Chapter XII BIOLOGICAL EFFECTS
INTRODUCTION
TYPES OF INJURIES
12.01 The three main types of physical effects associated with a nuclear explosion, namely, blast and shock, thermal radiation, and nuclear radiation, each have the potentiality for causing death and injury to exposed persons. Blast injuries may be direct or indirect; the former are caused by the high air pressure and the latter by missiles and by displacement of the body. For a given overpressure, a nuclear device is more effective in producing direct blast injuries than is a conventional, high-explosive weapon because, as will be seen, the human body is sensitive to the duration of the pressure pulse and this is relatively long in a nuclear explosion unless the yield is much less than 1 kiloton. On the whole, indirect blast injuries, especially those caused by missiles such as glass, wood, debris, etc., are similar for nuclear and conventional weapons. However, because of its longer duration, the blast wave from a nuclear explosion produces missile and displacement injuries at much lower overpressures than does a chemical explosion.
12.02 The frequency of burn injuries due to a nuclear explosion is exceptionally high. Most of these are flash burns caused by direct exposure to the pulse of thermal radiation, although individuals trapped by spreading fires may be subjected to flame burns. In addition, persons in buildings or tunnels close to ground zero may be burned by hot gases and dust entering the structure even though they are shielded adequately from direct or scattered thermal radiation. Finally, there are potential harmful effects of the nuclear radiations on the body. These represent a source of casualties entirely new to warfare.
12.03 A nuclear explosion in the air or near the ground will inevitably be accompanied by damage and destruction of buildings, by blast, shock, and fire, over a considerable area. Consequently, a correspondingly larger number of personal casualties is to be expected. However, the actual number, as well as their distribution among the different kinds of injuries mentioned above, will be greatly dependent upon circumstances. As a general rule, for bursts of a given type, e.g., air, surface, or sub surface, the range of each of the major immediate effects-blast, thermal radiation, and initial nuclear radiation-increases with the explosive yield of the weapon. But the relative importance of the various effects does not remain the same. The initial nuclear radiation, for example, is much more significant in comparison with blast and thermal radiation for nuclear explosions of low energy yield than it is for those of high yield. In other words, although the total number of casualties will increase with the energy of the explosion, under similar circumstances, the percentage of injuries due to initial nuclear radiation may be expected to decrease whereas the proportions of blast and thermal injuries will increase.
12.04 All other things, including exposure conditions, being the same, the number and distribution of casualties of various kinds for a nuclear explosion of given yield will be determined by the type of burst. Moreover, for an air burst, the height of burst will have an important influence. With other factors constant, there is an optimum height of burst which maximizes the range on the ground for a given overpressure in the blast wave (§ 3.73). This optimum height differs for each yield and for each value of the overpressure. Similarly, there are particular heights of burst, usually different from that for blast damage, which maximize the ranges for either thermal radiation or the initial nuclear radiation. It is evident, therefore, that considerable variations are possible both in the number and in the nature of the injuries associated with an air burst.
12.05 The effects of a surface or of a shallow subsurface burst will not be greatly different from those accompanying a low air burst. However, as increasing amounts of contaminated earth and debris are sucked up into the radioactive cloud the hazard from the residual nuclear radiation in the early fallout increases. For an underground burst at a moderate depth, the injuries from blast and from thermal and initial nuclear radiations would be much less than from an air burst or even from a surface burst of the same yield. On the other hand, the effects of ground shock and the delayed nuclear radiation hazard would be greatly increased. In the case of a deep (completely contained) underground burst, casualties would result only from ground shock.
12.06 Apart from the explosion yield and burst conditions, local environmental circumstances can be a significant factor in the casualty potential of a nuclear weapon. Conditions of terrain and weather can influence the injuries caused by blast and by thermal radiation. Structures may have an important, although variable, effect. For example, the shielding in ordinary houses may markedly reduce the range over which significant casualties from flash burns can occur. This is particularly the case for heavier structures extending below as well as above ground; persons properly located in such buildings could be protected from blast and initial nuclear radiations as well as from thermal radiations. On the other hand, in certain buildings the frequency of indirect blast injuries may be greatly increased by the presence of large numbers of missiles.
12.07 As regards direct injuries resulting from the overpressure of the air in the blast wave, the effects of a structure are also quite variable. In some situations it is known that the magnitude of the peak overpressure inside a structure can be appreciably less than the free-field (open terrain) value. On the other hand, there is a possibility that, as a result of reflection at walls, etc., the air overpressure in the interior of a building may be increased twofold or more, depending on the geometry involved (see Chapter IV). There will also be changes in wind velocity inside structures, so that the magnitudes may differ markedly from those existing in the free field as the blast wave spreads outward from the burst point. Nevertheless, provided people do not lean against the walls or sit or lie on the floor, there is generally a lower probability of injury from direct overpressure effects inside a structure than at equivalent distances on the outside. This results from alterations in the pattern of the overpressure wave upon entering the structure.
JAPANESE CASUALTIES
12.08 The only direct information concerning human casualties resulting from a nuclear explosion is that obtained following the air bursts over Japan and this will be used as the basis for much of the discussion presented here. It should be pointed out, however, that the Japanese experience applies only to the particular heights of burst and yields of the weapons exploded over Hiroshima and Nagasaki (§ 2.24), and to the weather, terrain, and other conditions existing at the times and locations of the explosions. Almost any kind of nuclear explosion in a populated area, except perhaps one deep under the surface, would be accompanied by a large number of deaths and injuries in a short interval of time, but the actual number of casualties and their distribution between blast (and shock), thermal, and nuclear radiation effects could vary markedly with the circumstances.
12.09 The data in Table 12.09 are the best available estimates1 for civilian casualties resulting from all effects of the explosions over Hiroshima and Nagasaki. The population estimates are only for civilians within the affected area in each city and do not include an unknown number of military personnel. Three zones, representing different distances from ground zero, are considered: the first is a circular area of 0.6 mile radius about ground zero, the second is a ring from 0.6 to 1.6 miles from ground zero, and the third is from 1.6 to 3.1 miles from ground zero. In each case there is given the total population in a particular zone, the population density, i.e., number per square mile, and the numbers of killed and injured, in that zone. Also included are the total population “at risk” in the city, the average population density, and the total numbers of killed and injured. The standardized casualty rates are values calculated by proportion on the basis of a population density of one person per 1,000 square feet (or about 28,000 per square mile) of vulnerable area.
Zone | Population | Density (per square mile) | Killed | Injured |
---|---|---|---|---|
Hiroshima |
||||
0 to 0.6 mile | 31,200 | 25,800 | 26,700 | 3,000 |
0.6 to 1.6 miles | 144,800 | 22,700 | 39,600 | 53,000 |
1.6 to 3.1 miles | 80,300 | 3,500 | 1,700 | 20,000 |
Totals | 256,300 | 8,500 | 68,000 | 76,000 |
Standardized Casualty Rate: 261,000 (Vulnerable area 9.36 square miles). | ||||
Nagasaki |
||||
0 to 0.6 mile | 30,900 | 25,500 | 27,300 | 1,900 |
0.6 to 1.6 miles | 27,700 | 4,400 | 9,500 | 8,100 |
1.6 to 3.1 miles | 115,200 | 5,100 | 1,300 | 11,000 |
Totals | 173,800 | 5,800 | 38,000 | 21,000 |
Standardized Casualty Rate: 195,000 (Vulnerable area 7.01 square miles). |
12.10 It is important to note that, although the average population densities in Hiroshima and Nagasaki were 8,500 and 5,800 per square mile, respectively, densities of over 25,000 per square mile existed in areas close to ground zero. For comparison, the average population density for the five boroughs of New York City, based on the 1970 census, is about 24,700 per square mile and for Manhattan alone it is 68,600 per square mile. The population density for the latter borough during the working day is, of course, much higher. The ten next largest U.S. cities have average population densities ranging from 14,900 to 3,000 persons per square mile.
12.11 The numbers in Table 12.09 serve to emphasize the high casualty potential of nuclear weapons. There are several reasons for this situation. In the first place, the explosive energy yield is very much larger than is possible with conventional weapons, so that both the area and degree of destruction are greatly increased. Second, because of the high energy yields, the duration of the overpressure (and winds) associated with the blastwave, for a given peak overpressure, is so long that injuries occur at overpressures which would not be effective in a chemical explosion. Third, the proportion of the explosive energy released as thermal radiation is very much greater for a nuclear weapon; hence there is a considerably larger incidence of flash burns. Finally, nuclear radiation injuries, which are completely absent from conventional explosions, add to the casualties.
12.12 The data in the table also show that more than 80 percent of the population within 0.6 mile (3170 feet) from ground zero were casualties. In this area the blast wave energy, thermal exposure, and initial nuclear radiation were each sufficient to cause serious injury or death. Beyond about 1.6 miles, however, the chances of survival were very greatly improved. Between 0.6 and 1.6 miles from ground zero a larger proportion of the population would probably have survived if immediate medical attention had been available. Although the particular distances mentioned apply to the yield and conditions of the Japanese explosions, it is to be expected quite generally that close to ground zero the casualty rate will be high, but it will drop sharply beyond a certain distance which scales with the energy yield of the explosion.
CAUSES OF FATALITIES
12.13 There is no exact information available concerning the relative significance of blast, burn, and nuclear radiation injuries as a source of fatalities in the nuclear bombings of Japan. It has been estimated that some 50 percent of the deaths were caused by burns of one kind or another, but this figure is only a rough estimate. Close to two-thirds of those who died at Hiroshima during the first day after the explosion were reported to have been badly burned. In addition, there were many deaths from burns during the first week.
12.14 The high incidence of flash burns caused by thermal radiation among both fatalities and survivors in Japan was undoubtedly related to the light and scanty clothing being worn, because of the warm summer weather prevailing at the time of the attacks. If there had been an appreciable cloud cover or haze below the burst point, the thermal radiation would have been attenuated somewhat and the frequency of flash burns would have been much less. Had the weather been cold, fewer people would have been outdoors and they would have been wearing more extensive clothing. Both the number of people and individual skin areas exposed to thermal radiation would then have been greatly reduced and there would have been fewer casualties from flash burns.
12.15 None of the estimates of the causes of death bear directly on the incidence of those blast effects which result in early death, e.g., air (emboli) in the arteries, lung damage, and heart injury which tolerate very little post-in jury activity, various bone fractures, severing of major blood vessels by sharp missiles, violent impact, and others. One of the difficulties in assessing the importance of injuries of various types lies in the fact that many people who suffered fatal blast injuries were also burned. As seen earlier, within about half a mile of ground zero in the Japanese explosions, either blast, burns, or nuclear radiation injury alone was lethal in numerous instances.
12.16 As a result of various circumstances, however, not everyone within a radius of half a mile was killed immediately. Among those who survived the first few days after the explosions at Hiroshima and Nagasaki, a number died two or more weeks later with symptoms which were ascribed to nuclear radiation injuries (see § 12.113 et seq.). These were believed to represent from 5 to 15 percent of the total fatalities. A rough estimate indicates that about 30 percent of those who died at Hiroshima had received lethal doses of nuclear radiation, although this was not always the immediate cause of death.
12.17 The death rate in Japan was greatest among individuals who were in the open at the time of the explosions; it was less for persons in residential (wood-frame and plaster) structures and least of all for those in concrete buildings. These facts emphasize the influence of circumstances of exposure on the casualties produced by a nuclear weapon and indicate that shielding of some type can be an important factor in survival. For example, within a range of 0.6 mile from ground zero over 50 percent of individuals in Japanese-type homes probably died of nuclear radiation effects, but such deaths were rare among persons in concrete buildings within the same range. The effectiveness of concrete structures in providing protection from injuries of all kinds is apparent from the data in Table 12.17; this gives the respective average distances from ground zero at which there was 50-percent survival (for at least 20 days) among the occupants of a number of buildings in Hiroshima. School personnel who were indoors had a much higher survival probability than those who were outdoors at the times of the explosions.
Approximate Distance (miles) | |
---|---|
Overall | 0.8 |
Concrete buildings | 0.12 |
School personnel: | |
Indoors | 0.45 |
Outdoors | 1.3 |
CAUSES OF INJURIES AMONG SURVIVORS
12.18 From surveys made of a large number of Japanese, a fairly good idea has been obtained of the distribution of the three types of injuries among those who became casualties but survived the nuclear attacks. The results are quoted in Table 12. 18; the totals add up to more than 100 percent, since many individuals suffered multiple injuries.
Injury | Percent of Survivors |
---|---|
Blast (mechanical) | 70 |
Burns (flash and flame) | 65 |
Nuclear radiation (initial) | 30 |
12.19 Among survivors the proportion of indirect blast (mechanical) injuries due to flying missiles and motion of other debris was smallest outdoors and largest in certain types of industrial buildings. Patients were treated for lacerations received out to 10,500 feet (2 miles) from ground zero in Hiroshima and out to 12,500 feet (2.2 miles) in Nagasaki. These distances correspond roughly to those at which moderate damage occurred to wood-frame houses, including the shattering of window glass.
12.20 An interesting observation made among the Japanese survivors was the relatively low incidence of serious mechanical injuries. For example, fractures were found in only about 4 percent of survivors. In one hospital there were no cases of fracture of the skull or back and only one fractured femur among 675 patients, although many such injuries must have undoubtedly occurred. This was attributed to the fact that persons who suffered severe concussion or fractures were rendered helpless, particularly if leg injuries occurred, and, along with those who were pinned beneath the wreckage, were trapped and unable to seek help or escape in case fire ensued. Such individuals, of course, did not survive.
CASUALTIES AND STRUCTURAL DAMAGE
12.21 For people who were in buildings in Japan, the overall casualties were related to the extent of structural damage, as well as to the type of structure (§ 12.17). The data in Table 12.21 were obtained from a study of 1,600 Japanese who were in reinforced-concrete buildings, between 0.3 and 0.75 mile from ground zero, when the nuclear explosions occurred. At these distances fatalities in the open ranged from about 90 to 100 percent, indicating, once more, that people were safer inside buildings, even when no special protective action was taken because of the lack of warning. There may have been an increase of casualties in buildings from debris etc., but this was more than compensated by the reduction due to shielding against the initial nuclear radiation and particularly from the thermal pulse.
Percent of Individuals | ||||
---|---|---|---|---|
Structural Damage | Killed Outright | Serious Injury (hospitalization) | Light Injury (no hospitalization) | No Injury Reported |
Severe damage | 88 | 44 | — | 1 |
Moderate damage | 14 | 18 | 21 | 47 |
Light damage | 8 | 14 | 27 | 51 |
12.22 In two concrete buildings closest to ground zero, where the mortality rate was 88 percent, about half the casualties were reported as being early and the other half as delayed. The former were attributed to a variety of direct and indirect blast injuries, caused by overpressure, structural collapse, debris, and whole-body translation, whereas the latter were ascribed mainly to burns and initial nuclear radiation. Minor to severe but nonfatal blast injuries no doubt coexisted and may have contributed to the delayed lethality in many cases. At greater distances, as the threat from nuclear radiation decreased more rapidly than did that from air blast and thermal radiation, the proportion of individuals with minor injuries or who were uninjured increased markedly. The distribution of casualties of different types in Japanese buildings was greatly influenced by where the people happened to be at the time of the explosion. Had they been forewarned and knowledgeable about areas of relative hazard and safety, there would probably have been fewer casualties even in structures that were badly damaged.
12.23 The shielding effect of a particular building is not only different for blast, the thermal pulse, and nuclear radiation, but it may also depend on the distance from the explosion and the height of the burst. Furthermore, the locations and orientations of individuals in the building are important in determining the extent of the shielding. Hence, the protection offered by structures is quite variable. This fact must be kept in mind in considering the data in Table 12.21. Although the table indicates a general correlation between structural damage and the frequency of casualties, the numbers cannot be used to estimate casualties from the degree of structural damage. In an actual situation, the effects would depend on many factors, including the type of structure, the yield of the nuclear explosion, the height of the burst, the distance from the explosion point, the locations and orientations of people in the building, and the nature of prior protective action.
BLAST INJURIES
DIRECT BLAST INJURIES: BIOLOGICAL FACTORS
12.24 Blast injuries are of two main types, namely, direct (or primary) injuries associated with exposure of the body to the environmental pressure variations accompanying a blast wave, and indirect injuries resulting from impact of penetrating and nonpenetrating missiles on the body or as the consequences of displacement of the body as a whole. There are also miscellaneous blast injuries, such as burns from the gases and debris, and irritation and possibly suffocation caused by airborne dust. The present section will treat direct injuries, and indirect blast effects will be discussed later.
12.25 The general interactions of a human body with a blast wave are somewhat similar to that of a structure as described in Chapter IV. Because of the relatively small size of the body, the diffraction process is quickly over, the body being rapidly engulfed and subjected to severe compression. This continues with decreasing intensity for the duration of the positive phase of the blast wave. At the same time the blast wind exerts a drag force of considerable magnitude which contributes to the displacement hazard.
12.26 The sudden compression of the body and the inward motion of the thoracic and abdominal walls cause rapid pressure oscillations to occur in the air-containing organs. These effects, together with the transmission of the shock wave through the body, produce damage mainly at the junctions of tissues with air-containing organs and at areas between tissues of different density, such as where cartilage and bone join soft tissue. The chief consequences are hemorrhage and occasional rupture of abdominal and thoracic walls.
12.27 The lungs are particularly prone to hemorrhage and edema (accumulation of fluid causing swelling), and if the injury is severe, air reaches the veins of the lungs and hence the heart and arterial circulation. Death can occur in a few minutes from air embolic obstruction of the vessels of the heart or the brain or from suffocation caused by lung hemorrhage or edema. Fibrin emboli in the blood may also affect the brain and other critical organs. The emboli, apparently associated with severe hemorrhagic damage to the lungs, are a consequence of the disturbance of the blood-clotting mechanism. Damage to the brain due to air blast overpressure alone is improbable, but indirect damage may arise from injury to the head caused by missiles, debris, or displacement of the body. Bodily activity after blast damage to the heart and lungs is extremely hazardous and lethality can result quickly where recovery might otherwise have been expected. The direct blast effect was not specifically recognized as a cause of fatality in Japan, but it no doubt contributed significantly to early mortality even though most of the affected individuals may also have received mortal injury from debris, displacement, fire, or thermal and nuclear radiations.
12.28 Primary blast casualties have been reported after large-scale air at tacks with conventional high-explosive bombs, mainly because of the provision of medical care for those who otherwise would have suffered the early death that is characteristic of serious blast injury to the lungs. However, persons who spontaneously survive for 24 to 48 hours in the absence of treatment, complications, and other injury usually recover and show little remaining lung hemorrhage after 7 to 10 days. In very severe injuries under treatment, recurring lung hemorrhage has been reported as long as 5 to 10 days after injury. In view of such facts and overwhelming disruptive effects of the Japanese bombings on med ical and rescue services, it can be concluded that individuals with significant direct blast injuries did not survive. Those with relatively minor blast injuries who did survive, did so without getting into medical channels, or if they did require medical care it was for post blast complications, e.g., pneumonitis, or for causes other than blast injury to the lungs. For these reasons primary blast effects, except for eardrum rupture, were not commonly seen among Japanese survivors.
12.29 Many persons who apparently suffered no serious injury reported temporary loss of consciousness. This symptom can be due to the direct action of the blast wave, resulting from transient disturbance of the blood circulation in the brain by air emboli. However, it can also be an indirect effect arising from impact injury to the head caused by missiles or by violent displacement of the body by the air pressure wave.
12.30 A number of cases of ruptured eardrums were reported among the survivors in Hiroshima and Nagasaki, but the incidence was not high even for those who were fairly close to ground zero. Within a circle of0.31 mile (1,640 feet) radius about 9 percent of a group of 44 survivors in Nagasaki had ruptured eardrums, as also did some 8 percent of 125 survivors in the ring from 0.31 to 0.62 mile from ground zero. In Hiroshima the incidence of ruptured eardrums was somewhat less. In both cities very few cases were observed beyond 0.62 mile.
DIRECf BLAST INJURIES: PHYSICAL FACfORS
12.31 Tests with animals have demonstrated that five parameters of the blast wave can affect the extent of the direct injuries to the body; they are (I) the ambient pressure, (2) the “effective” peak overpressure, (3) the rate of pressure rise (or “rise time”) at the blast wave front, (4) the character and “shape” of the pressure pulse, and (5) the duration of the positive phase of the blast wave and the associated wind (see Chapter III). These parameters will be considered below as they arise.
12.32 The biologically effective peak overpressure depends on the orientation of the individual to the blast wave. If the subject is against a reflecting surface, e.g., a wall, the effective overpressure for direct blast injury is equal to the maximum reflected over pressure, which may be a few times the incident peak overpressure. On the other hand, in the open at a substantial distance from a reflecting surface, the effective overpressure is the sum of the peak incident overpressure and the associated peak dynamic pressure if the subject is perpendicular to the direction of travel of the blast wave and to the peak overpressure alone if the subject is parallel to this direction. Consequently, for a given incident overpressure, the blast injury is expected to be greatest if the individual is close to a wall and least if he is at a distance from a reflecting surface and is oriented with his body parallel to the direction in which the blast wave is moving.
12.33 The body, like many other structures, responds to the difference between the external and internal pressures. As a consequence, the injury caused by a certain peak overpressure depends on the rate of increase of the pressure at the blast wave front. For wave fronts with sufficiently slow pressure rise, the increase in internal pressure due to inward movement of the body wall and air flow in the lungs keeps pace (to some extent) with the external pressure. Consequently, quite high incident overpressures are tolerable. In contrast, if the rise time is short, as it is in nuclear explosions under appropriate terrain and burst conditions, the damaging effect of a given overpressure is greater. The increase in internal pressure of the body takes a finite time and the response is then to the maximum possible pressure differential. Thus, a sharply rising pressure pulse will be more damaging than if the same peak overpressure is attained more slowly. In precursor formation (§ 3.79 et seq.), for example, the blast pressure increases at first slowly and then quite rapidly; the injury potential of a given peak overpressure is thus decreased.
12.34 An individual inside a building but not too close to a wall would be subject to multiple reflections of the blast wave from the ceiling, floor, and walls as well as to the incident wave entering the structure. Since the reflected waves would reach him at different times, the result would be a step loading, although the rise time for each step might be quite short. In such cases, where the initial blast pressure is tolerable and the subsequent pressure increase is not too great or occurs in stages (or slowly), a certain peak overpressure is much less hazardous than if it were applied in a single sharp pulse. Apparently the reason for the decreased blast injury potential in these situations is that the early stage of the pressure pulse produces an increase in the internal body pressure, thereby reducing the pressure differential associated with the later portion of the pulse. In a manner of speaking, a new and higher “ambient” pressure is imposed on the body by the early part of the pressure pulse and tolerance to the later rise in overpressure is enhanced. A higher peak overpressure is then required to cause a certain degree of blast injury.
12.35 Clearly, for a given peak incident overpressure, the geometry2 in which an individual is exposed inside a structure may have a significant effect on his response to air blast. A location against a wall is the most hazardous position because the effective peak overpressure, which is the maximum reflected overpressure, is high and is applied rapidly in a single step. A location a few feet from a wall is expected to decrease the direct blast injury, although the hazard arising from displacement of the body may be increased. Apart from the effects just described, oscillating pressures, for which no adequate bio medical criteria are available, often exist inside structures due to reverberating reflections from the inside walls.
12.36 The duration of the positive phase of the blast wave is a significant factor for direct blast injuries. Up to a point, the increase in the duration increases the probability of injury for a given effective peak overpressure. Beyond this point, which may be of the order of several tens to a few hundred milliseconds, depending on the body size, 1t 1s only the magnitude of the overpressure that is important. The duration of the positive phase, for a given peak overpressure, varies with the energy yield and the height of burst (§ 3.75 et seq.). But for most conditions, especially for energy yields in excess of about 10 kilotons, the duration of the positive phase of the blast wave is so long-approaching a second or more-that the effective peak overpressure is the main factor for determining the potential for direct injury from a fast-rising pressure pulse.
12.37 A given peak pressure in the blast wave from conventional high explosives is less effective than from a nuclear explosion--except perhaps at unusually low yields-mainly because of the short duration of the positive phase in the former case. From observations made with small charges of chemical explosives, it has been estimated that deaths in humans would require sharp-rising effective overpressures as high as 200 to 400 (or more) pounds per square inch when the positive phase durations are less than a millisecond or so. These pressures may be compared with values of roughly 50 (or less) to about 100 pounds per square inch, with positive phase durations of the order of a second, for nuclear explosions.
12.38 Tentative criteria, in terms of effective peak overpressure as defined in § 12.32, for lung damage, lethality, and eardrum rupture caused by a fast-rising pressure pulse of long duration (O.1 second or more) are given in Table 12.38. The values for lung damage and lethality are average pressures obtained by extrapolation from animal data to man; the variability of the results is indicated by the numbers in parentheses. Rupture of the normal eardrum is apparently a function of the age of the individual as well as of the effective blast pressure. Failures have been recorded at overpressures as low at 5 pounds per square inch ranging up to 40 or 50 pounds per square inch. The values in Table 12.38 of the effective peak overpressures for eardrum rupture are based on relatively limited data from man and animals.
Effect | Effective Peak Pressure (psi) |
---|---|
Lung Damage: | |
Threshold | 12 ( 8-15) |
Severe | 25 (20-30) |
Lethality: | |
Threshold | 40 (30-50) |
50 percent | 62 (50-75) |
100 percent | 92 (75-115) |
Eardrum Rupture: | |
Threshold | 5 |
50 percent | 15-20 (more than 20 years old) |
30-35 (less than 20 years old) |
INDIRECT BLAST INJURIES
12.39 Indirect blast injuries are associated with (1) the impact of missiles, either penetrating or nonpenetrating (secondary effects), and (2) the physical displacement of the body as a whole (tertiary effects). The wounding potential of blast debris depends upon a number of factors; these include the impact (or striking) velocity, the angle at which impact occurs, and the size, shape, density, mass, and nature of the moving objects. Furthermore, consideration must be given to the portion of the body involved in the missile impact, and the events which may occur at and after the time of impact, namely, simple contusions and lacerations, at one extreme, or more serious penetrations, fractures, and critical damage to vital organs, at the other extreme.
12.40 The hazard from displacement depends mainly upon the time and distance over which acceleration and deceleration of the body occur. Injury is more likely to result during the latter phase when the body strikes a solid object, e.g., a wall or the ground. The velocity which has been attained before impact is then significant. This is determined by certain physical parameters of the blast wave, as mentioned below, as well as by the orientation of the body with respect to the direction of motion of the wave. The severity of the damage depends on the magnitude of the impact velocity, the properties of the impact surface, and the particular portion of the body that has received the decelerative impact, e.g., head, back, extremities, thoracic and abdominal organs, body wall, etc.
DISPLACEMENT VELOCITIES
12.41 Because the effects of both missiles and body displacement depend on the velocity attained before impact, it is convenient to consider the relationships between displacement velocity and the blast parameters for objects as small as tiny pieces of glass and as large as man. The significant physical factors in all cases are the magnitude and duration of the blast overpressure and the accompanying winds, the acceleration coefficient of the displaced object,3 ground shock, gravity, and the distance traveled by the object. The latter is important because, as a result of the action of the blast wave, the velocity of the object increases with the time and distance of travel until it attains that of the blast wind. Subsequently, the velocity falls because of negative winds or impact with the ground or other material.
12.42 As a result of the interaction of the various factors, large and heavy objects gain velocity rather slowly and attain a maximum velocity only after most of the blast wave has passed. The velocity is consequently determined by the duration of the overpressure and winds. In contrast, small and light objects reach their maximum velocity fairly quickly, often after a small proportion of the blast wave has passed over them. The maximum velocity is thus not too sensitive to the duration of the overpressure and winds, but depends largely on the effective peak overpressure (cf. § 12.32). As a consequence of this fact, it has been found possible to relate the velocities attained by the fragments produced by the breakage of glass window panes to the effective overpressure. The results for glass panes of different thicknesses can be expressed in a fairly simple graphical manner as will be shown in § 12.238.
12.43 The variations of the overpressure and dynamic pressure with time (§ 3.57 et seq.) at the location of interest also have a bearing on the behavior of a displaced object. Data were obtained at nuclear weapons tests under such conditions that the blast wave was approximately ideal in behavior. Some of the median velocities, masses, and spatial densities (number of fragments per square foot) of window glass, from houses exposed to the blast, and of natural stones are summarized in Table 12.43 For glass, the velocities refer to those attained after 7 to 13 feet of travel; for the stones the distances are not known, but the velocities given in the table may be regarded as applicable to optimum distances of missile travel.
Missile | Peak Overpressure (psi) | Median Velocity (ft/sec) | Median Mass (grams) | Maximum Number per Sq Ft |
---|---|---|---|---|
Glass | 1.9 | 108 | 1.45 | 4.3 |
Glass | 3.8 | 168 | 0.58 | 159 |
Glass | 3.9 | 140 | 0.32 | 108 |
Glass | 5.0 | 170 | 0.13 | 388 |
Stones | 8.5 | 286 | 0.22 | 40 |
12.44 Studies have also been made of the displacement of anthropomorphic dummies weighing 165 pounds by the blast from a nuclear explosion. A dummy standing with its back to the blast attained its maximum velocity, about 21 feet per second, after a displacement of 9 feet within 0.5 second after the arrival of the blast wave. The free-field overpressure at the test location was 5.3 pounds per square inch. The dummy traveled 13 feet before striking the ground and then slid or rolled another 9 feet. A prone dummy, however, did not move under the same conditions. The foregoing results were obtained in a situation where the blast wave was nearly ideal, but in another test, at a peak overpressure of 6.6 pounds per square inch, where the blast wave was non ideal (§ 3.79), both standing and prone dummies suffered considerably greater displacements. Even in such circumstances, however, the displacement of over 125 feet for the prone dummy was much less than that of about 250 feet for the standing one. The reason for the greater displacement of the standing dummy is that it acquired a higher velocity.
12.45 In order to study the displacements of moving objects, field tests have been made by dropping animal cadavers, including guinea pigs, rabbits, goats, and dogs, and stones and concrete blocks onto a flat, hard surface from a vehicle traveling between 10 and 60 miles per hour (14.7 to 88 feet per second). For a given initial velocity, the stopping distance for the animals increased somewhat with the mass, and a relationship was found to represent the stopping distance as a function of velocity applicable to the animals over a wide range of mass (§ 12.239). One reason for the consistency of the data is probably that all the animals assumed a rolling position about their long axis regardless of the initial orientation. The animals remained relatively low to the ground and bounced very little. By contrast, stones and concrete blocks bounced many times before stopping; the data were not sensitive to mass, depended more on orientation, and were more variable than the results obtained with animals. On the whole, the stopping distances of the blocks and stones were greater for a given initial velocity. One of the conclusions drawn from the foregoing tests was that a person tumbling over a smooth surface, free from rocks and other hard irregularities, might survive, even if the initial velocity is quite high, if he could avoid head injury and did not flail his limbs.
MISSILE AND DISPLACEMENT INJURY CRITERIA
12.46 Velocity criteria for the production of skin lacerations by penetrating missiles, e.g., glass fragments, are not known with certainty. Some reliable information is available, however, concerning the probability of penetration of the abdominal wall by glass. The impact velocities, for glass fragments of different masses, corresponding to 1, 50, and 99 percent penetration probability are recorded in Table 12.46.
Probability of Penetration (percent) | |||
---|---|---|---|
Mass of Glass Fragments (grams) | |||
1 | 50 | 99 | |
Impact Velocity (ft/sec) | |||
0.1 | 235 | 410 | 730 |
0.5 | 160 | 275 | 485 |
1.0 | 140 | 245 | 430 |
10.0 | 115 | 180 | 355 |
12.47 The estimated impact velocities of a 10-gram (0.35-ounce) glass missile required to produce skin lacerations and serious wounds are summarized in Table 12.47. The threshold value for skin lacerations is recorded as 50 feet per second and for serious wounds it is 100 feet per second.
Effect | Impact Velocity (ft/sec) |
---|---|
Skin laceration: | |
Threshold | 50 |
Serious wounds: | |
Threshold | 100 |
50 percent | 180 |
Near 100 percent | 300 |
12.48 Little is known concerning the relationship between mass and velocity of nonpenetrating missiles that will cause injury after impact with the body. Studies with animals showed that fairly high missile velocities are required to produce lung hemorrhage, rib fractures, and early mortality, but quantitative data for man are lacking. No relationship has yet been developed between mass and velocity of nonpenetrating missiles that will cause injury as a result of impacts with other parts of the body wall, particularly near the spine, kidney, liver, spleen and pelvis. It appears, however, that a missile with a mass of 10 pounds striking the head at a velocity of about 15 feet per second or more can cause skull fracture. For such missiles it is unlikely that a significant number of dangerous injuries will occur at impact velocities of less than 10 feet per second. The impact velocities of a 10-pound missile for various effects on the head are given in Table 12.48.
Effect | Impact Velocity (ft/sec) |
---|---|
Cerebral Concussion: | |
Mostly “safe” | 10 |
Threshold | 15 |
Skull Fracture: | |
Mostly “safe” | 10 |
Threshold | 13 |
Near 100 percent | 23 |
In this connection, a casualty is defined as an individual so injured that he would probably be a burden on others. Some of the casualties would prove fatal, especially in the absence of medical care.
12.49 Although there may be some hazard associated with the accelerative phase of body displacement (translation) by a blast wave, the deceleration, particularly if impact with a solid object is involved, is by far the more significant. Since a hard surface will cause a more serious injury than a softer one, the damage criteria given below refer to perpendicular impact of the displaced body with a hard, flat object. From various data it is concluded that an impact velocity of 10 feet per second is unlikely to be associated with a significant number of serious injuries; between 10 and 20 feet per second some fatalities may occur if the head is involved; and above 20 feet per second, depending on trauma to critical organs, the probabilities of serious and fatal injuries increase rapidly with increasing displacement velocity. Impact velocities required to produce various indirect (tertiary) blast effects are shown in Table 12.49. The curves marked “translation near structures” in Fig. 12.49 may be used to estimate ground distances at which 1 percent and 50 percent casualties would be expected, as functions of height of burst, for a 1-kiloton explosion.5 Based on tests with animals, the criteria for 1 and 50 percent casualties were somewhat arbitrarily set at impact velocities of 8 and 22 feet per second, respectively. The results in Fig. 12.49 may be extended to other burst heights and yields by using the scaling law given in the example facing the figure.
Effect | Impact Velocity (ft/sec) |
---|---|
Standing Stiff Legged Impact: | |
Mostly “safe” | |
No significant effect | < 8 |
Severe discomfort | 8-10 |
Injury | |
Threshold | 10-12 |
Fracture threshold (heels, feet, and legs) | 13-16 |
Seated Impact: | |
Mostly “safe” | |
No significant effect | < 8 |
Severe discomfort | 8-14 |
Injury | |
Threshold | 15-26 |
Skull Fracture: | |
Mostly “safe” | 10 |
Threshold | 13 |
50 percent | 18 |
Near 100 percent | 23 |
Total Body Impact: | |
Mostly “safe” | 10 |
Lethality threshold | 21 |
Lethality 50 percent | 54 |
Lethality near 100 percent | 138 |
12.50 Evaluation of human tolerance to decelerative tumbling during translation in open terrain is more difficult than for impact against a rigid surface described above. Considerably fewer data are available for decelerative tumbling than for body impact, and there is virtually no human experience for checking the validity of extrapolations from observations on animal cadavers. Tests have been made with goats, sheep, and dogs, but for humans the information required to derive reliable hazards criteria for decelerative tumbling is still not adequate. The initial velocities at which 1 and 50 percent of humans are expected to become casualties as a result of decelerative tumbling have been tentatively estimated to be 30 and 75 feet per second, respectively. The curves in Fig. 12.49 marked “translation over open terrain” are approximate, but they may be used to provide a general indication of the range within which casualties might occur from decelerative tumbling due to air blast from surface and air bursts.
BURN INJURIES
CLASSIFICATION OF BURNS
12.51 Thermal radiation can cause burn injuries either directly, i.e., by absorption of the radiant energy by the skin, or indirectly by heating or ignition of clothing, or as a result of fires started by the radiation. The direct burns are often called “flash burns,” since they are produced by the flash of thermal radiation from the fireball. The indirect (or secondary) burns are referred to as “contact burns” or “flame burns”; they are identical with skin burns that result from touching a hot object or those that would accompany (or be caused by) any large fire no matter what its origin. In addition, individuals in buildings or tunnels close to ground zero may be burned from hot debris, gases, and dust (§ 12.02).
12.52 A skin burn is an injury caused by an increase in skin temperature resulting from direct absorption of thermal radiation, which varies with skin color, or from the transference of heat through clothing. The severity of the burn depends on the amount of the temperature increase and on the duration of the increase. For example, a skin temperature of 70°C (155°F) for a fraction of a second will produce the same type of burn as a temperature of 48°C (118°F) for a few minutes. Skin burns are generally classified as first, second, or third degree, in order of increasing severity of the burn. Pain associated with skin burns occurs when the temperature of certain nerve cells near the surface is raised to 43°C ( 109°F) or more. If the temperature is not sufficiently high or does not persist for a sufficient length of time, pain will cease and no injury will occur. The amount of pain is not directly related to the severity of the burn injury, but it can serve a useful purpose in warning an individual to evade part of the thermal pulse from a nuclear explosion.
12.53 First-degree burns, which are the mildest, are characterized by immediate pain and by ensuing redness of the affected area. The pain continues even after the temperature of the skin has returned to normal. The first-degree burn is a reversible injury; that is to say, healing is complete with no scar formation. Sunburn is the classic example of first-degree burn.
12.54 Second-degree burns result from skin temperatures that are higher and/or of longer duration than those causing first-degree skin burns. The injury is characterized by pain which persists, and may be accompanied either by no immediate visible effect or by a variety of skin changes including blanching, redness, loss of elasticity, swelling, and development of blisters. After 6 to 24 hours, a scab will form over the injured area. The scab may be flexible and tan or brown, if the injury is moderate, or it may be thick, stiff, and dark, if the injury is more severe. The wounds will heal within one to two weeks unless they are complicated by infection. Second-degree burns do not involve the full thickness of the skin, and the remaining uninjured cells may be able to regenerate normal skin without scar formation.
12.55 If skin temperatures become sufficiently high and/or are of long duration, third-degree burns will be produced. Pain is experienced at the peripheral, less injured areas only, since the nerve endings in the centrally burned areas are damaged to the extent that they are unable to transmit pain impulses. Immediately after suffering the burn, the skin may appear either normal, scalded, or charred, and it may lose its elasticity. The healing of third-degree burns takes several weeks and will always result in scar formation unless new skin is grafted over the burned area. The scar results from the fact that the full thickness of the skin is injured, and the skin cells are unable to regenerate normal tissue.
12.56 The distribution of burns into three groups obviously has certain limitations since it is not possible to draw a sharp line of demarcation between first and second-degree, or between second and third-degree burns. Within each class the burn may be mild, moderate, or severe, so that upon preliminary examination it may be difficult to distinguish between a severe burn of the second degree and a mild third-degree burn. Subsequent pathology of the injury, however, will usually make a distinction possible. In the following discussion, reference to a particular degree of burn should be taken to imply a moderate burn of that type.
12.57 The depth of the burn is not the only factor in determining its effect on the individual. The extent of the area of the skin which has been affected is also important. Thus, a first-degree burn over the entire body may be more serious than a third-degree burn at one spot. The larger the area burned, the more likely is the appearance of symptoms involving the whole body. Furthermore, there are certain critical, local regions, such as the hands, where almost any degree of burn will incapacitate the individual.
12.58 Persons exposed to nuclear explosions of low or intermediate yield may sustain very severe burns on their faces and hands or other exposed areas of the body as a result of the short pulse of directly absorbed thermal radiation. These burns may cause severe superficial damage similar to a third-degree burn, but the deeper layers of the skin may be uninjured. Such burns would heal rapidly, like mild second-degree burns. Thermal radiation burns occurring under clothing or from ignited clothing or other tinder will be similar to those ordinarily seen in burn injuries of nonnuclear origin. Because of the longer duration of the thermal pulse from an air burst weapon in the megaton range, flash burns on exposed skin and burns of nonnuclear origin may also be similar.
BURNS UNDER CLOTHING
12.59 Skin burns under clothing, which depend on the color, thickness, and nature of the fabric, can be produced in the following ways: by direct transmittance through the fabric if the latter is thin and merely acts as an attenuating screen; by heating the fabric and causing steam or volatile products to impinge on the skin; by conduction from the hot fabric to the skin; or the fabric may ignite and hot vapors and flames will cause burns where they impinge on the skin. Burns beneath clothing can arise from heat transfer for some time after the thermal pulse ends. These burns generally involve deeper tissues than the flash burns produced by the direct thermal pulse on bare skin. Flame burns caused by ignited clothing also result from longer heat application, and thus will be more like burns due to conventional conflagrations.
12.60 First- and second-degree burns of the uncovered skin and burns through thin clothing occur at lower radiant exposures (§ 7.35) than those which ignite clothing (Table 7.36). Because of these factors, first- and second-degree burns in exposed persons would involve only those body areas that face the explosion. Where the direct thermal pulse produces third-degree burns and clothing ignition takes place, persons wearing thin clothing would have such burns over parts of the body facing the burst. Persons wearing heavy clothing could suffer third-degree burns over the whole body if the ignited clothing could not be removed quickly. This phenomenon is typically seen in persons whose clothing catches fire by conventional means.
INCAPACITATION FROM BURNS
12.61 Burns of certain areas of the body, even if only of the first degree, will frequently result in incapacitation because of their critical location. Any burn surrounding the eyes that causes occluded vision, e.g., because of swelling of the eyelids, will be incapacitating. Burns of the elbows, knees, hands, and feet produce immobility or limitation of motion as the result of swelling, pain, or scab formation, and will cause ineffectiveness in most cases. The occurrence of burns of the face, neck, and hands are probable because these areas are most likely to be unprotected. Second- or third-degree burns in excess of 20 percent of the surface area of the body should be considered major burns and will require special medical care in a hospital. If the nose and throat are seriously involved and obstructive edema (§ 12.27) occurs, breathing may become impossible and tracheotomy may be required as a life-saving measure.
12.62 Shock is a term denoting a generalized state of serious circulatory inadequacy. If serious, it will result in incapacitation and unconsciousness and if untreated may cause death. Third-degree burns of 25 percent of the body and second-degree burns of 30 percent of the body will generally produce shock within 30 minutes to 12 hours and require prompt medical treatment. Such treatment is complicated and causes a heavy drain on medical personnel and supply resources.
RADIANT EXPOSURES FOR BURNS ON EXPOSED SKIN
12.63 The critical radiant exposure for a skin burn depends on the duration of the radiation pulse and the thermal energy spectrum; both of these quantities vary with the yield and height of burst. Hence, although the radiant exposure is known as a function of distance and yield (see Chapter VII), it is not a simple matter to predict distances at which burns of different types may be expected from a given explosion. Apart from radiant exposure, the probability and severity of the burns will depend on several factors. One of the most important is the absorptive properties of the skin for thermal radiation. In a normal population, the fraction of the radiation energy absorbed may vary by as much as 50 percent because of differences in skin pigmentation.
12.64 For thermal radiation pulses of 0.5 second duration or more, as is the case for explosions with yields exceeding 1-kiloton, the energy absorbed by the skin, rather than the radiant exposure, determines the extent of the burn injury. The spectral absorptance of the skin, i.e., the fraction of the incident radiation energy (or radiant exposure) that is absorbed, depends on the skin pigmentation. The curves in Fig. 12.64 have been derived from thermal energy spectra of nuclear explosions in the lower part of the atmosphere and measured values of the absorptance of different skin types. By considering explosions in the lower atmosphere, the height of the burst variable is largely eliminated. The results in the figure are applicable to exposed skin when no evasive action is taken and there is no protection from structures or clothing. It is seen that the radiant exposure required to produce a given degree of burn injury varies significantly with skin pigmentation. In fact, people with very dark skins could receive burns from approximately two-thirds the incident radiant energy that will cause similar burns in very light-skinned people.
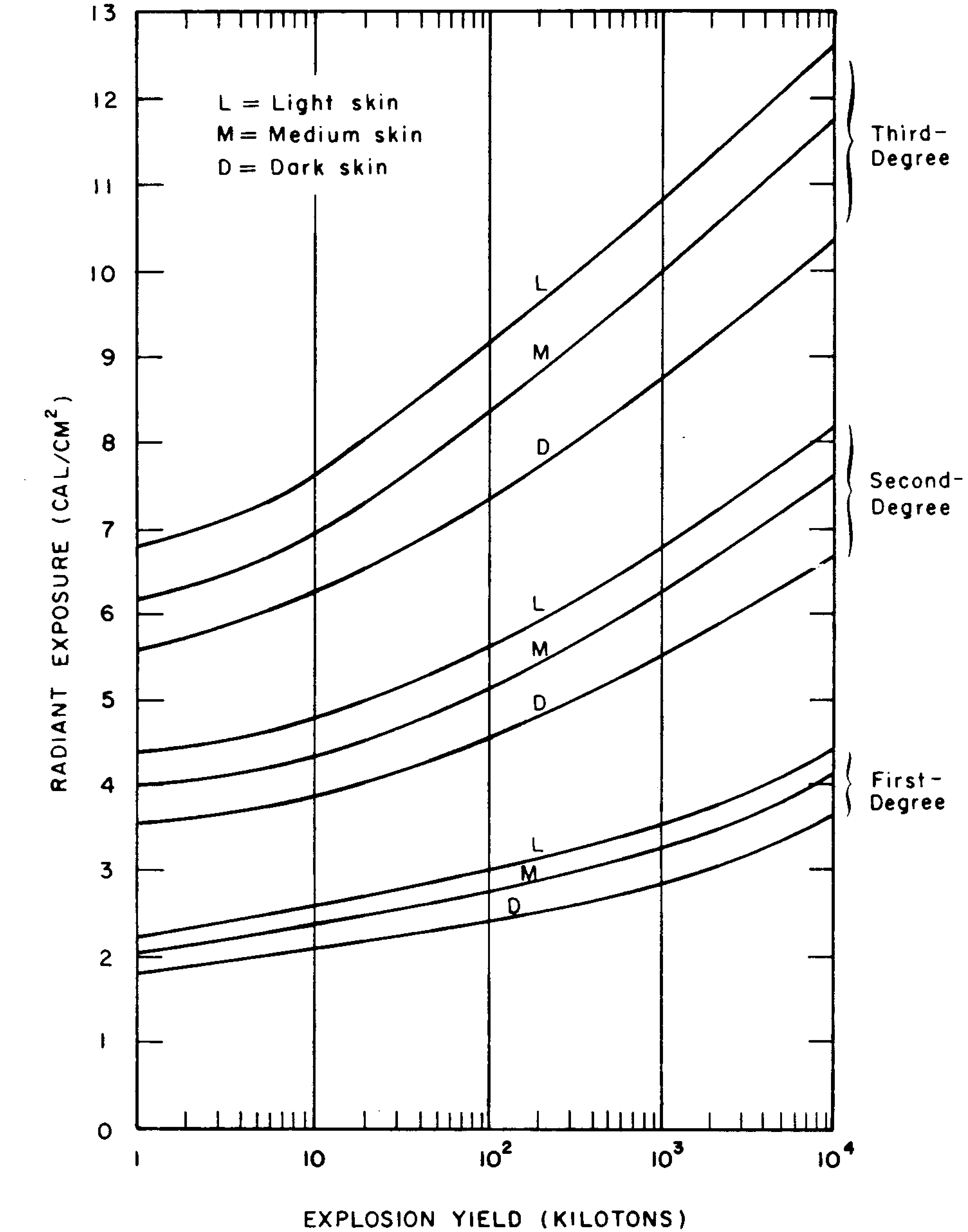
12.65 Figure 12.65 shows radiant exposures for the various probabilities of burn occurrence, again assuming no evasive or protective action. The solid lines represent the conditions under which it is probable that 50 percent of an average exposed population will receive skin burns of the indicated degree. The broken lines divide the burn probability distributions into ranges for three degrees of burn severity with average probabilities of 18 percent and 82 percent assigned within the various ranges. For example, from Fig. 12.65 it is expected that, if a normal population is exposed to the thermal pulse from a 1-megaton explosion in the lower atmosphere, at distances where the radiant exposures are between 4.5 and 6 cal/cm2, 18 percent of the population will receive second-degree burns and the remainder first-degree burns to the exposed (un protected) skin.
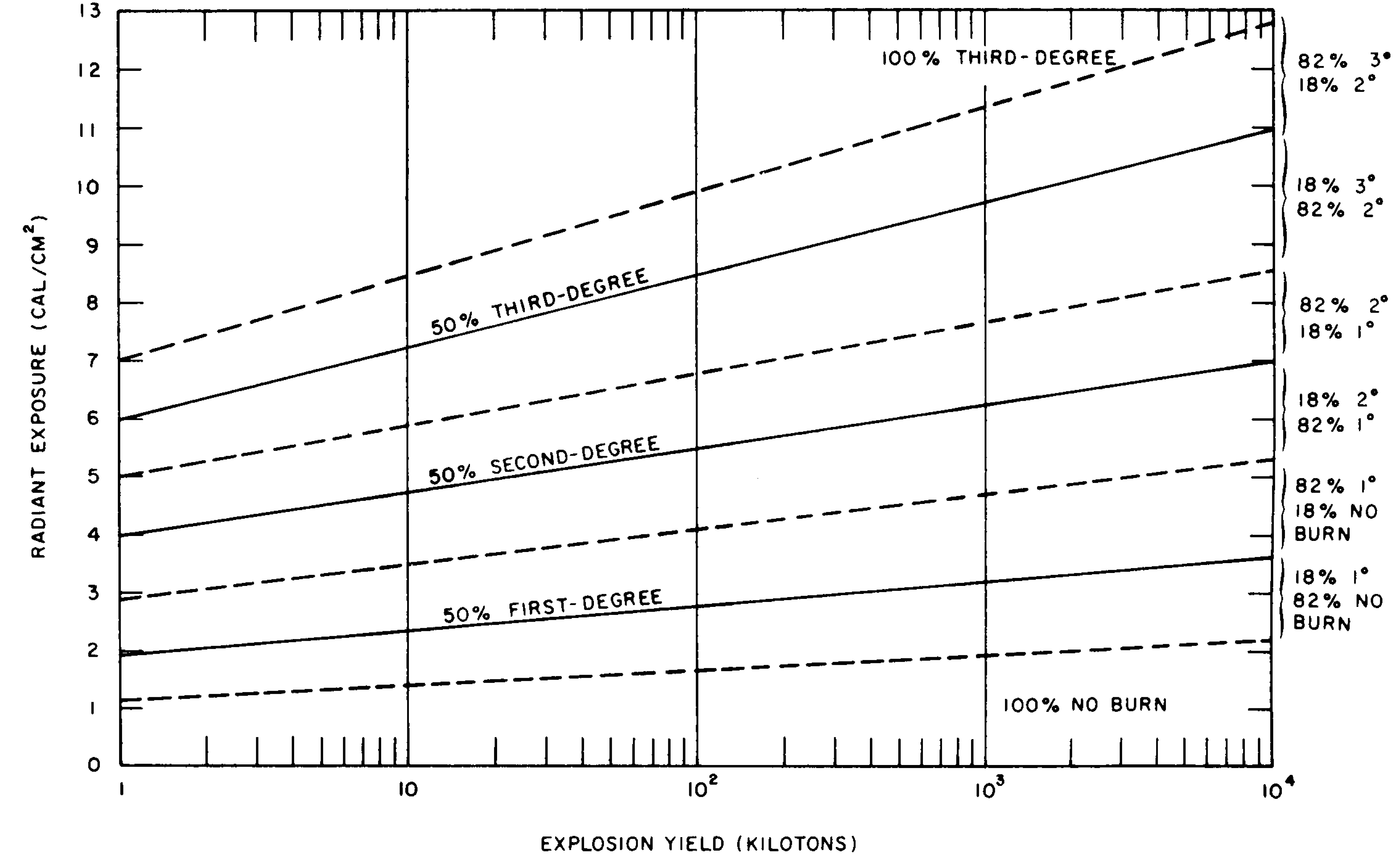
12.66 With the aid of the yield distance relationships for various radiant exposures given in Chapter VII, the curves in Fig. 12.65 may be used to determine the approximate distances from ground zero at which given burn probabilities may be experienced. Suppose that, in the example given above, the 1-megaton weapon is detonated at a height of 10,000 feet, which is within the lower atmosphere. According to Fig. 7.42, for air bursts below 20,000 feet and 12-mile visibility, the specified radiant exposure between 4.5 and 6 cal/cm2, would be received at slant ranges of from 9 to 10 miles. Since these ranges are substantially greater than the height of burst (about 2 miles), they may be taken as the distances to ground zero to the accuracy of Fig. 7.42. Hence, within the radii of 9 and 10 miles from ground zero, it is probable that 18 percent of an average population subjected to the whole thermal pulse will receive second-degree burns and 82 percent first-degree burns to their exposed (unprotected) skin.
12.67 As already noted, the burn criteria given above are based on the supposition that no evasive action is taken. For air bursts with yields less than about 100 kilotons, the main part of the thermal energy arrives too quickly for people to react and take some protective action. Evasion of part of the thermal energy that would be effective in reducing burn injuries is possible, however, for yields of 100 kilotons or more in the lower atmosphere. The length of the thermal pulse is then such that the pain could initiate a reaction which, if appropriate, might allow a person to obtain sufficient protection to decrease the severity of the potential burn (§ 7.87). The ability to react in this manner can apparently be improved by appropriate training.
BURN INJURIES IN JAPAN
12.68 Among the survivors of the nuclear explosions in Japan, the incidence of flame burns appeared to be very small. In fact, they constituted not more than 5 percent of the total burn injuries. This was the case because most of those who suffered flame burns did not survive, since they were caught in burning buildings and could not escape. The character of the flame burns was similar to that of burns caused by other conflagrations. The clothing usually caught fire and then large parts of the body suffered flame burns. By contrast, as will be seen below, flash burns were generally restricted to exposed skin areas, i.e., face, arms, hands, and legs.
12.69 One of the most striking consequences of the nuclear bombings of Japan was the large number of casualties due to flash burns caused by the thermal radiation. The situation was aggravated by the clear atmosphere and warm weather which prevailed at the time (§ 12.14). It was estimated that 20 to 30 percent of the fatal casualties in Hiroshima and Nagasaki were caused by flash burns. In the former city alone, about 42,000 burn cases were reported and of those some 24,500 were recorded as being serious. Unless protected by heavy clothing, thermal radiation burns, apart from other injuries, would have been fatal to nearly all unshielded persons in the open at distances up to 6,000 feet (1.1 miles) or more from ground zero. Even as far out as 12,000 to 14,000 feet (2.3 to 2.6 miles), there were instances of such burns which were bad enough to require treatment.
THERMAL RADIATION BURNS IN JAPAN
12.70 A distinctive feature of the thermal radiation (flash) burns was their sharp limitation to exposed areas of the skin facing the center of the explosion. For this reason they are sometimes called “profile burns” (Fig. 12.70). The phenomenon occurred because most of the radiation received had traveled in a straight line from the fireball and so only regions that were directly exposed were affected. A striking illustration of this behavior was that of a man writing before a window. His hands were seriously burned, but his face and neck, which were not covered, suffered only slight burns because the angle of entry of the thermal radiation through the window was such as to place them in partial shadow.
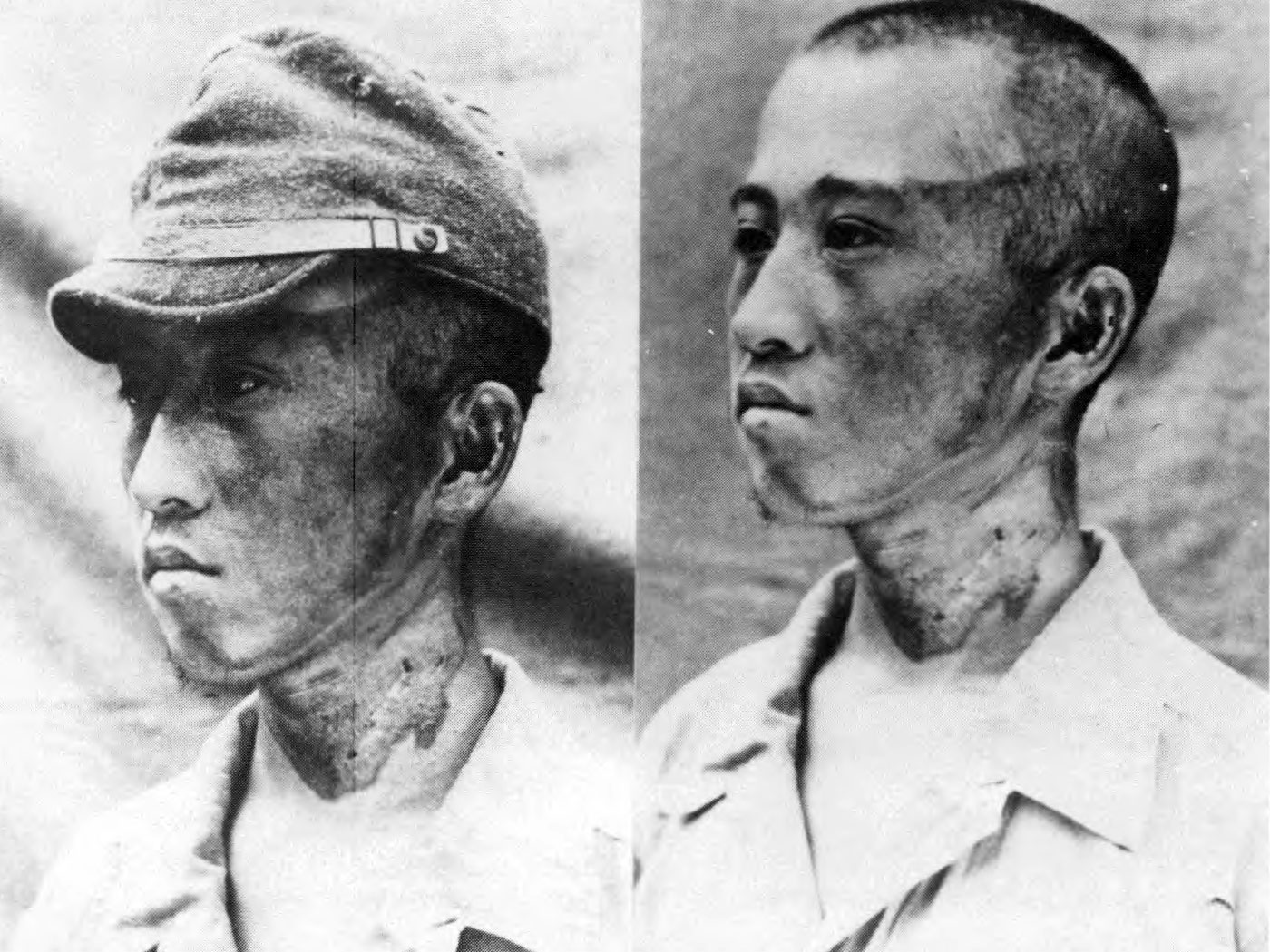
12.71 Although flash burns were largely confined to exposed parts of the body, there were a few cases where such burns occurred through one, and very occasionally more, layers of clothing. Instances of this kind were observed when the radiant exposure was large enough to overcome the protective effect of the particular fabric. When burns did occur through clothing, they frequently involved regions where the clothes were in contact with the skin, at the elbows and shoulders, for example. Such burns may have been due to heat transmitted from the hot fabric, rather than to the direct effect of radiation. Areas over which the clothing fitted loosely, so that an air space separated it from the skin, were generally unharmed by the thermal radiation (Fig. 12.71).
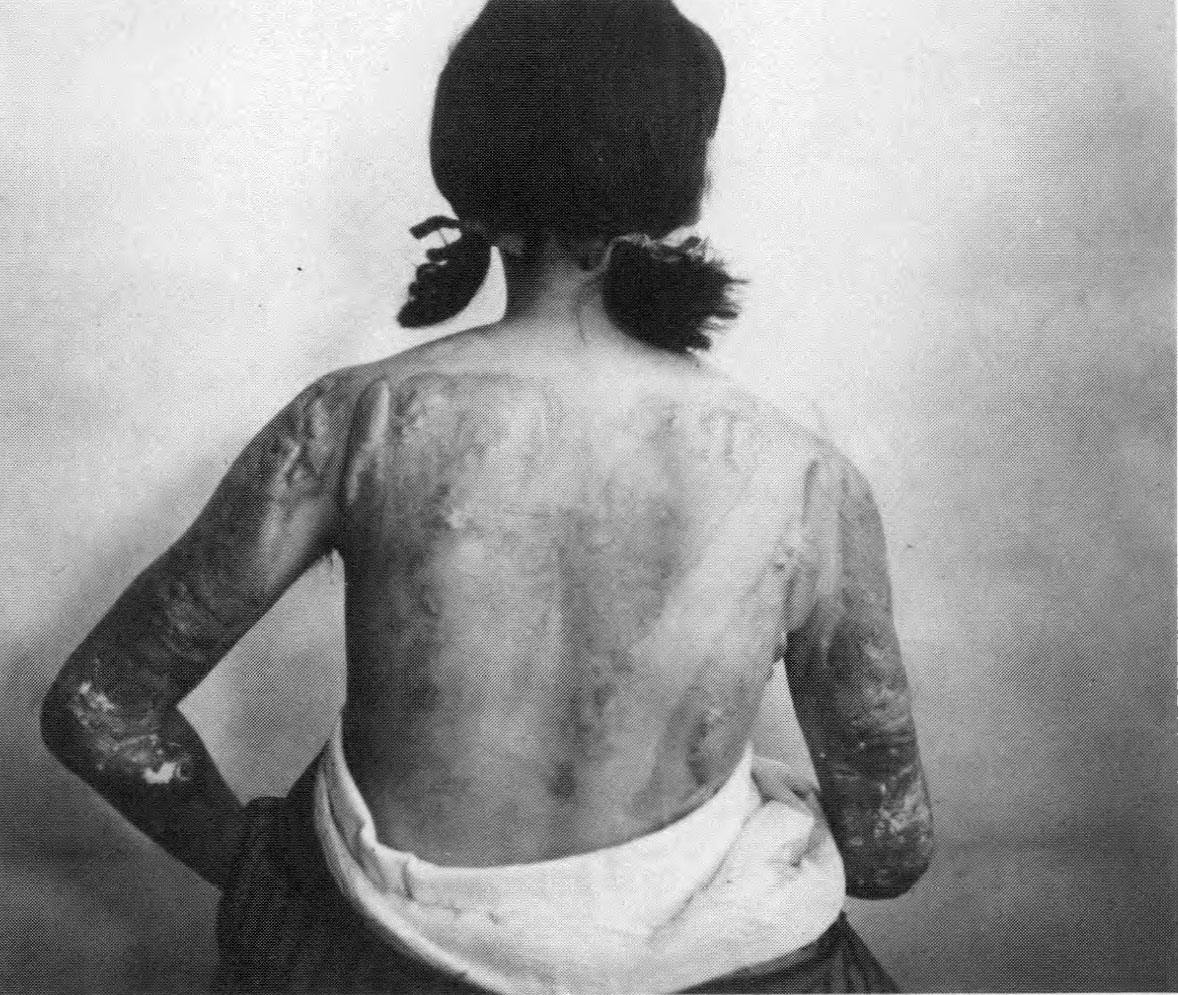
12.72 There were many instances in which burns occurred through black clothing, but not through white material worn by the same individual (Fig. 12.72). This was attributed to the reflection of thermal radiation by white or other light-colored fabrics, whereas materials of dark color absorbed radiation, became hot, and so caused contact burns. In some cases black outer clothing actually burst into flame and ignited the undergarments, so that flame burns resulted. It should be mentioned, however, that white clothing does not always necessarily provide protection against thermal radiation. Some materials of this kind transmit enough radiation to permit flash burning of the skin to occur.
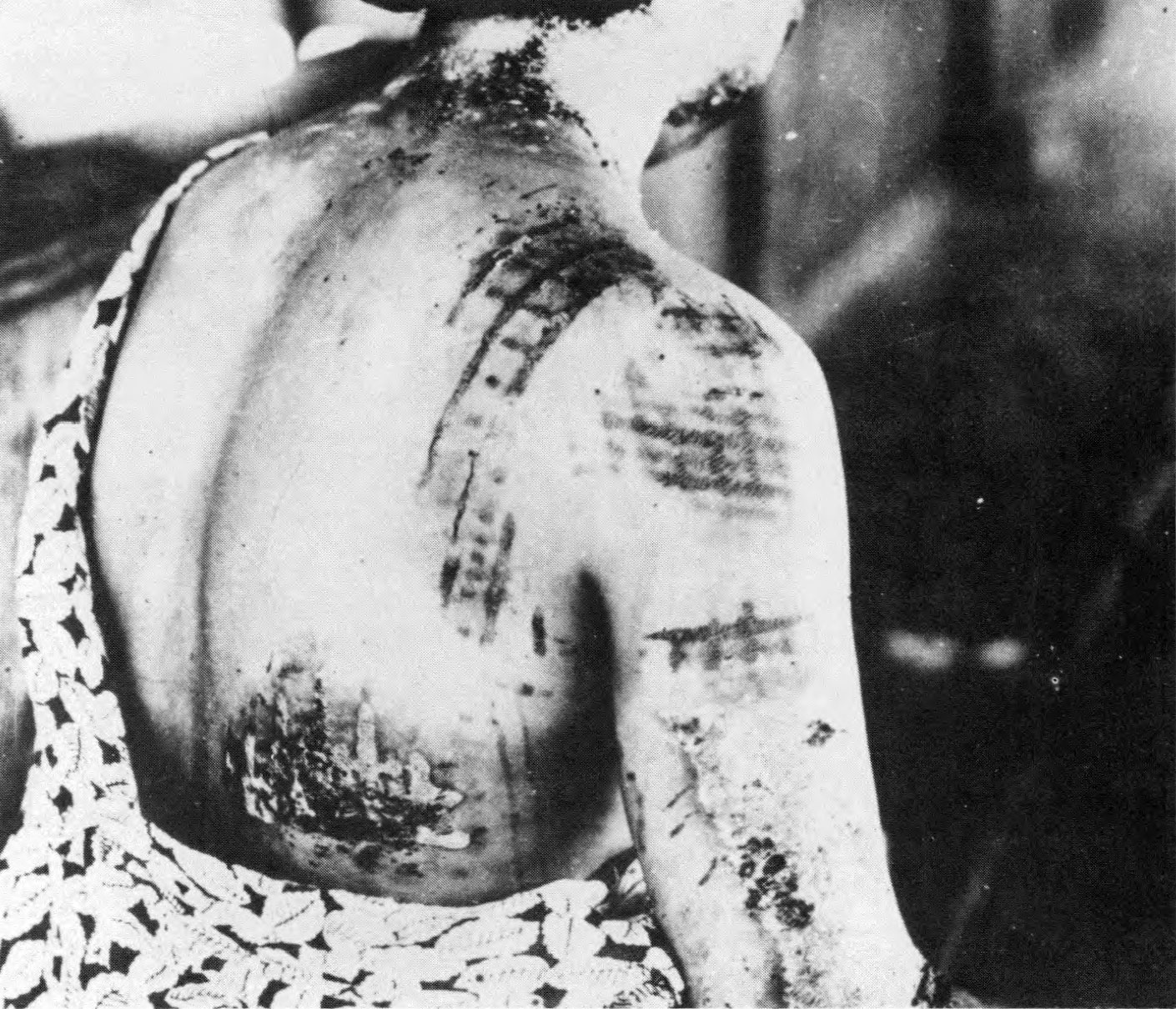
12.73 The frequency of flash burns was, of course, greatest among persons who were in the open. Nevertheless, there were a surprising number of such burns among individuals who were in doors. This was largely because many windows, especially in commercial structures, were uncurtained or were wide open on account of the summer weather. Hence, many persons inside buildings were directly exposed to thermal radiation. In addition to the protection afforded by clothing, particularly if light in color, some shielding was provided by the natural promontories of the body, e.g., the nose, supraorbital (eye socket) ridges, and the chin.
GENERAL CHARACTERISTICS OF FLASH BURNS
12.74 In spite of the thousands of flash burns experienced after the nuclear attacks on Japan, only their general features were reported. However, this information has been supplemented by observations made, especially on anesthetized pigs, both in the laboratory and at nuclear test explosions. The skin of white pigs has been found to respond to thermal radiation in a manner which is in many respects similar to, and can be correlated with, the response of human skin.
12.75 Severity of the flash burns in Japan ranging from mild erythema (reddening) to charring of the outermost layers of the skin. Among those who were within about 6,000 feet (1.1 miles) from ground zero, the bur injuries were depigmented lesions (light in color), but at greater distances, from 6,000 to 12,000 feet (1.1 to 2.3 miles), the initial erythema was followed by the development of a walnut coloration of the skin, sometimes called the “mask of Hiroshima.”
12.76 Burns of moderate second degree (and milder) usually healed within four weeks, but more severe burns frequently became infected so that the healing process was much more prolonged. Even under the best conditions, it is difficult to prevent burns from becoming infected, and after the nuclear bombings of Japan the situation was aggravated by inadequate care, poor sanitation, and general lack of proper facilities. Nuclear radiation injury may have been a contributory factor in some cases because of the decrease in resistance of the body to infection.
12.77 Experimental flash burns have been produced both in the laboratory and in nuclear tests which were apparently quite similar to those reported from Hiroshima and Nagasaki. In the more severe cases of circular experimental burns there was a central charred region with a white outer ring surrounded by an area of erythema. A definite demarcation both in extent and depth of the burns was noted, so that they were unlike contact burns which are generally variable in depth. The surface of the flash burns became dry without much edema or weeping of serum.
12.78 Another phenomenon, which appeared in Japan after the healing of some of the more severe burns, was the formation of keloids, that is, thick overgrowths of scar tissue. It was suggested, at one time, that they might have been due to nuclear radiation, but this view is no longer accepted. The degree of keloid formation appears to have been influenced by infections, which complicated healing of the burns, and by malnutrition. A secondary factor is the known disposition for keloid formation to occur among the Japanese and other dark-skinned people as a racial characteristic. Many spectacular keloids, for example, were formed after the healing of burns produced in the incendiary bomb attacks on Tokyo. There is a tendency, however, for keloids to disappear gradually in the course of time.
EFFECTS OF THERMAL RADIATION ON THE EYES
12.79 It is of interest that, among the survivors in Hiroshima and Nagasaki, eye injuries directly attributable to thermal radiation appeared to be relatively unimportant. There were many instances of temporary blindness, occasionally lasting up to 2 or 3 hours, but only one case of retinal injury was reported.
12.80 The eye injury known as keratitis (an inflammation of the cornea) occurred in some instances. The symptoms, including pain caused by light, foreign-body sensation, lachrymation, and redness, lasted for periods ranging from a few hours to several days. Among 1,000 cases, chosen at random, of individuals who were in the open, within some 6,600 feet (1.25 miles) of ground zero at the time of the explosions, only 42 gave a history of keratitis coming on within the first day. Delayed keratitis was reported in 14 additional cases, with symptoms appearing at various times up to a month or more after the explosion. It is possible that nuclear radiation injury, which is associated with delayed symptoms, as will be seen below, may have been a factor in these patients.
12.81 Investigators have reported that in no case, among 1,400 examined, was the thermal radiation exposure of the eyes apparently sufficient to produce permanent opacity of the cornea. This observation is not surprising since the cornea is transparent to the major portion of the thermal energy which is received in the visible and longer wavelength (infrared) parts of the spectrum. In approximately one-quarter of the cases studied there had been facial burns and often singeing of the eyebrows and eyelashes. Nevertheless, some 3 years later the corneas were found to be normal.
12.82Several reasons have been suggested for the scarcity of severe eye injuries in Japan. For example, the detonations occurred in the morning in broad daylight when the eye pupil would be expected to be small. Another possible explanation is that the recessed position of the eyes and, in particular, the overhanging upper lids served to decrease the direct exposure to thermal radiation. Furthermore, on the basis of probability, it is likely that only a small proportion of individuals would be facing the explosions in such a way that the fireball would actually be in their field of vision.
12.83 Exposure of the eye to the bright flash of a nuclear detonation can produce two possible injuries: flash blindness and retinal burns. Flashblindness (dazzle) is a temporary impairment of vision caused by a bleaching of the light-sensitive elements (rods and cones) in the retina of the eye. It may be produced by scattered light and does not necessarily require the eye to be focused on the fireball. Flashblindness will normally blank out the entire visual field of view with a bright afterimage. The effects persist only a short time and recovery is complete.
12.84 During the period of flash blindness (several seconds to minutes) useful vision is lost. This may preclude effective performance of activities requiring constant, precise visual function. The severity and time required for recovery of vision are determined by the intensity and duration of the flash, the viewing angle from the burst, the pupil size, brightness of the object being viewed and its background, and the visual complexity of the object. Flash blindness would be more severe at night since the pupil is larger and the objects and background are usually dimly illuminated.
12.85 A retinal burn is a permanent eye injury that occurs whenever the retinal tissue is heated excessively by the. image of the fireball focused in the eye. The underlying pigmented cells absorb much of the light (radiation) energy and the temperature is increased in that area. A temperature elevation of 12 to 20°C (22 to 36°F) in the eye produces a thermal injury that involves both the pigmented layer and the adjacent rods and cones, so that visual capacity is permanently lost in the burned area. The natural tendency of people to look directly at the fireball would increase the incidence of retinal burns. A retinal burn normally will not be noticed by the individual concerned if it is off the central axis of vision, but very small burned areas may be noticeable if they are centrally located. A person generally will be able to compensate for a small retinal burn by learning to scan around the burned area.
12.86 Retinal burns can be produced at great distances from nuclear detonations, because the probability of their occurrence does not decrease as the square of the distance from the detonation, as is true of many other nuclear weapons effects. Theoretically, the optical process of image formation within the eye should keep the energy per unit area on the retina constant, regardless of the distance. However, meteorological conditions and the fact that the human eye is not a perfect lens, all contribute toward reducing the retinal burn hazard as the distance is increased between the observer and the detonation.
12.87 Explosions with yields of more than about I megaton at heights greater than some 25 miles may produce retinal burns as far out as the horizon on clear nights. If the burst height is greater than some 50 miles, the short pulse of thermal energy from the early-time weapon debris, as well as that from the X-ray pancake, can be effective :n this respect (§ 7.91). Bursts above 90 miles altitude probably will not cause retinal burns in persons on the ground, unless the yield is greater than 10 megatons. The eye's blink reflexes are sufficiently fast (roughly 0.25 second) to provide some protection against weapons of more than 100 kilotons yield detonated below about 25 miles. The blink time is too slow to provide any appreciable protection from smaller weapons or from bursts at higher altitudes. When people have adequate warning of an impending nuclear burst, evasive action, including closing or shielding the eyes, will prevent both flashblindness and retinal burns.
12.88 Safe separation distances from ground zero, i.e., distances beyond which persons on the ground would not receive incapacitating eye in juries, are shown in Figs. 12.88a and b as a function of weapon yield for two heights of burst (HOB). The curves in Fig. 12.88a are for a clear day; for a cloudy day the safe separation distances would be reduced to about half. The curves in Fig. 12.88b are for night conditions. The distances for retinal burns are those for which such burns will not occur provided the eye can blink within 0.25 second. A faster blink time will not change the values appreciably, but a slower time would increase them. Data for the complete absence of flashblindness are not available and the distances in Figs. 12.88a and b are those within which a visual loss for about 10 seconds may be expected, to a degree sufficient to preclude the performance of a precision task under conditions of dim light, e.g., a pilot reading instruments at night.
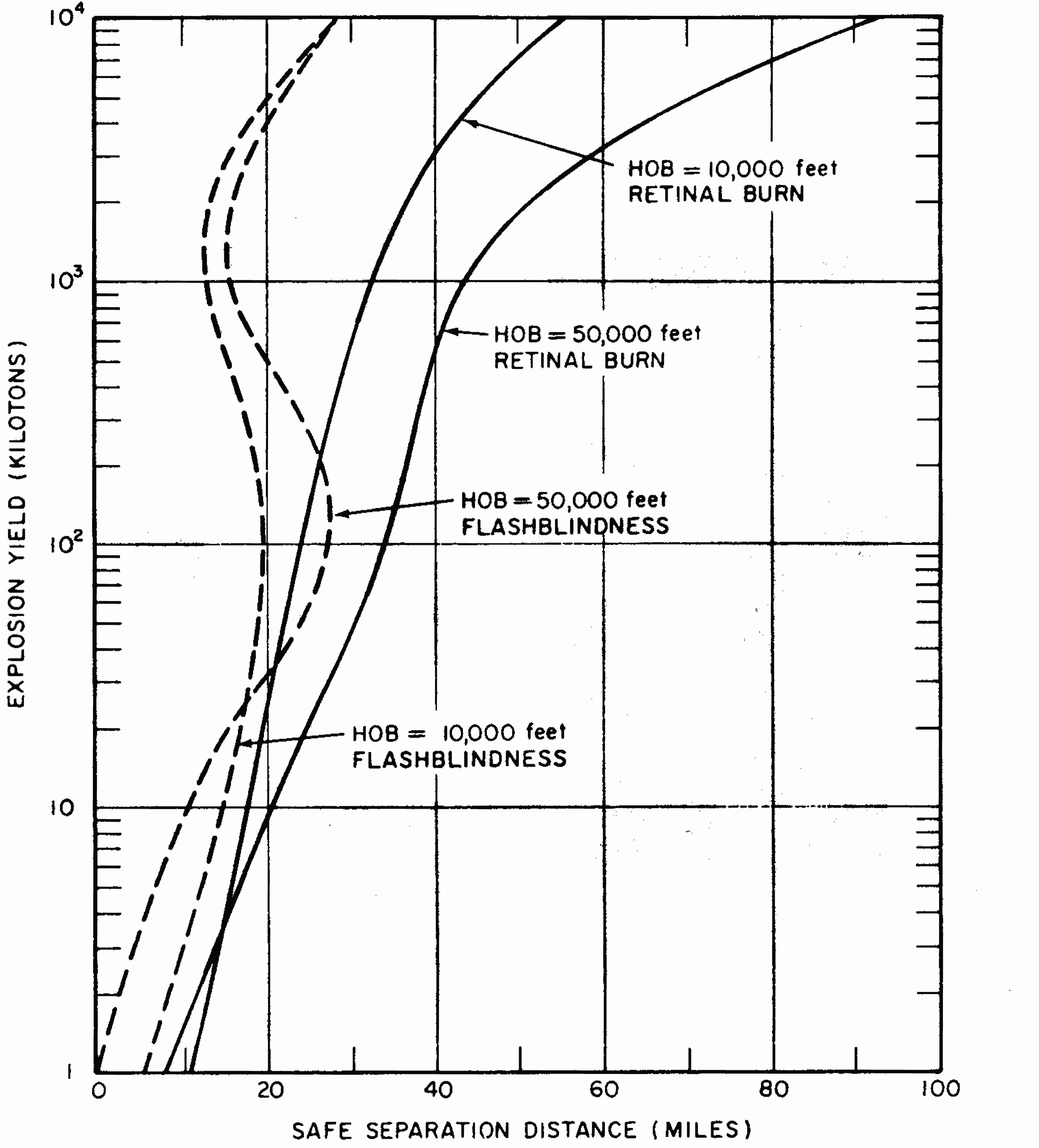
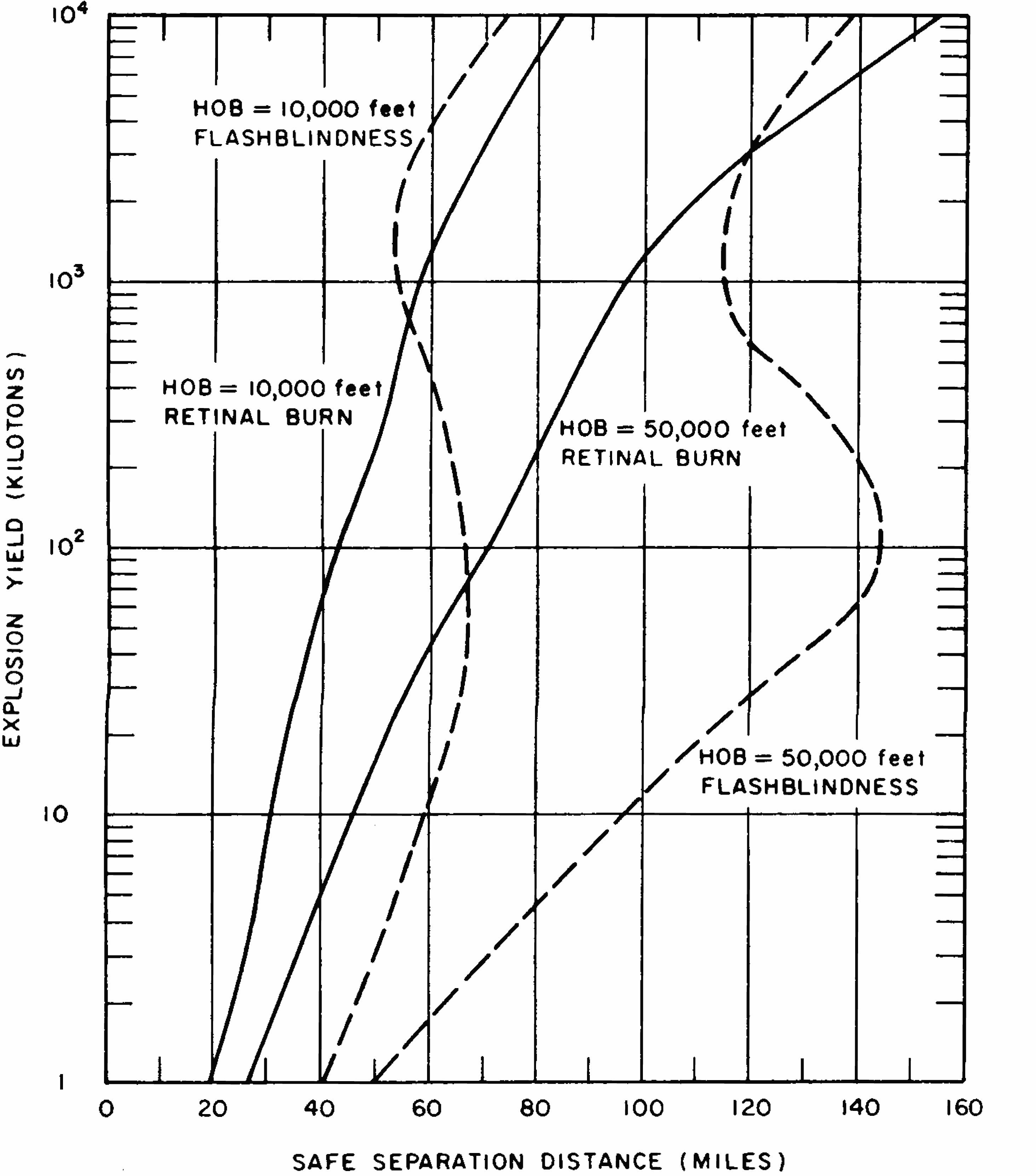
12.89 The flashblindness and retinal burn safe separation distances do not bear a constant relationship to one another as the yield changes. In circumstances that require determination of complete eye safety (bearing in mind the 10-second visual loss criterion for flashblindness), the effect that occurs at the greater distance from the burst is the critical one. For example, for a height of burst of 50,000 feet at night, it is seen from Fig. 12.88b that for yields up to about 3 megatons, flashblindness is the important factor in determining the distance at which there will be no. incapacitating eye effects. For larger yields, retinal burn becomes the limiting factor. Where only permanent eye damage is of interest and the temporary loss of vision from flashblindness is of little concern, the retinal burn curves should be used to estimate safe distances no matter what the explosion energy yield.
NUCLEAR RADIATION INJURY
INTRODUCTION
12.90 The injurious effects of nuclear radiations from a nuclear explosion represent a phenomenon which is completely absent from conventional explosions. For this reason, the subject of radiation injury (or sickness) will be described at some length. It should be understood, however, that the extended discussion does not necessarily imply that nuclear radiation would be the most important source of casualties in a nuclear explosion. This was certainly not the case in Japan where the detonations occurred at heights of approximately 1,870 feet (Hiroshima) and 1,640 feet (Nagasaki) above the ground. Such injuries as were caused by nuclear radiation were due to the initial radiation. The effect of the residual radiation, in the form of early fallout and induced radioactivity, was negligible. However, as was seen in Chapter IX, the situation could be very different in the event of a surface burst.
12.91 It has long been known that exposure to radiations, such as X rays, alpha and beta particles, gamma rays, and neutrons, which are capable of producing ionization, either directly or indirectly (§§ 8.21, 8.58), can cause injury to living organisms. After the discovery of X rays and radioactivity, toward the end of the nineteenth century, it became increasingly apparent that an element of danger was associated with exposure to ionizing radiations.6 In spite of the growing awareness by both scientists and physicians of the hazards inherent in many radiation sources, there were some excessive exposures. In the course of time, however, recommendations for preventing overexposure were adopted and radiation injuries became less frequent. Nevertheless, occasional overexposures have occurred among personnel operating radiographic equipment, powerful X-ray machines in industrial laboratories and hospitals, cyclotrons, and experimental nuclear reactors, or working with radioactive materials.
12.92 The harmful effects of nuclear radiations appear to be caused by the ionization (and excitation) produced in the cells composing living tissue. As a result of ionization, some of the constituents, which are essential to the normal functioning of the cells, are altered or destroyed. In addition, the products formed may act as poisons. Among the observed consequences of the action of ionizing radiations on cells are breaking of the chromosomes, swelling of the nucleus and of the entire cell, increase in viscosity of the cell fluid, increased permeability of the cell membrane, and destruction of cells. In addition, the process of cell division (or “mitosis”) is delayed by exposure to radiation. Frequently, the cells are unable to undergo mitosis, so that the normal cell replacement occurring in the living organism is inhibited.
RADIATION DOSE UNITS
12.93 The radiation unit known as the roentgen was described in § 8.17. By definition, it is applicable only to gamma rays or X rays. and not to other types of ionizing radiation, such as alpha and beta particles and neutrons. Since the roentgen refers to a specific result in air accompanying the passage of an amount of radiation through the air, it does not imply any effect that it would produce in a biological system. The roentgen is thus a measure of the “exposure” to gamma rays and X rays. The effect on a biological system, such as the whole body or a particular organ, however, depends on the amount of radiation energy that has been absorbed by the body or organ. The unit of absorbed dose, which applies to all kinds of ionizing radiations, including alpha and beta particles and neutrons, is the rad, as defined in § 8.18.
12.94 Although all ionizing radiations are capable of producing similar biological effects, the absorbed dose (measured in rads) which will produce a certain effect may vary appreciably from one type of radiation to another. This difference in behavior is expressed by means of the “relative biological effectiveness” (or RBE) of the particular nuclear radiation. The RBE of a given radiation is defined as the ratio of the absorbed dose in rads of gamma radiation (of a specified energy)7 to the absorbed dose in rads of the given radiation having the same biological effect. The value of the RBE for a particular type of nuclear radiation depends upon several factors, including the dose rate, the energy of the radiation, the kind and degree of the biological damage, and the nature of the organism or tissue under consideration.
12.95 The “biological dose,” also called the “RBE dose,” that provides a direct indication of the expected effects of any ionizing radiation on the body (or organ), is stated in terms of the “rem,” an abbreviation of “roentgen equivalent (in) man.” It is equal to the absorbed dose in rads multiplied by the RBE for the particular radiation (or radiations) absorbed; thus,
Dose in rems = Dose in rads x RBE.
An advantage of the rem is that it is possible to express the total biological effect that might result from the absorption of more than one kind of ionizing radiation. The absorbed dose in rads of each radiation type is multiplied by the appropriate RBE and the results are added. (In connection with radiological protection in peacetime activities, the “dose equivalent” in rems is defined as the absorbed dose in rads multiplied by a “quality factor,” and sometimes by other modifying factors. The quality factor, which depends on the nature and energy of the absorbed radiation, replaces the RBE.)
12.96 All radiations capable of producing ionization (or excitation) directly or indirectly, e.g., alpha and beta particles, X rays, gamma rays, and neutrons, cause radiation injury of the same general type. Although the effects are qualitatively similar, the various radiations differ in the depth to which they penetrate the body and in the degree of injury corresponding to a specified amount of energy absorption. As seen above, the latter difference is expressed by means of the RBE.
12.97 The RBE for gamma rays is approximately unity, by definition, although it varies somewhat with the energy of the radiation. For beta particles, the RBE is also close to unity; this means that for a given amount of energy absorbed in living tissue, beta particles produce about the same extent of injury within the body as do X rays or gamma rays.8 The RBE for alpha particles from radioactive sources that have been taken into the body is in the range from 10 to 20, more specifically for the development of bone cancers. The RBE for neutrons varies with the energy and the type of injury. For the neutron energy spectrum of nuclear weapons, the RBE for immediate (acute) radiation injury is close to 1.0. But it is significantly larger (4 to 10) for the occurrence of opacities of the eye lens (cataracts), leukemia, and genetic changes (§ 12.144 et seq., § 12.201 et seq.). For these biological effects, a certain amount of energy absorbed from exposure to neutrons is much more damaging than the same amount of energy (in rads) absorbed from gamma rays.9
GENERAL CHARACTERISTICS OF RADIATION EFFECTS
12.98 In considering the possible effects on the body of ionizing radiations from external sources, it is necessary to distinguish between an “acute” (or “one-shot”) exposure and a “chronic” (or extended) exposure. In an acute exposure the whole radiation dose is received in a relatively short interval of time. This is the case, for example, in connection with the initial nuclear radiation. It is not possible to define an acute dose precisely, but it may be somewhat arbitrarily taken to be the dose received during a 24-hour period. Although the delayed radiations from early fallout persists for longer times, the main exposure would be received during the first day and so it is regarded as being acute. On the other hand, an individual entering a fallout area after the first day or so and remaining for some time would be considered to have been subjected to a chronic exposure.
12.99 The importance of making a distinction between acute and chronic exposures lies in the fact that, if the dose rate is not too large, the body can achieve partial recovery from many (but perhaps not all) of the consequences of nuclear radiations. For example, an acute dose of 50 rems will generally cause changes in the constituents of the blood (§ 12.113), but the same dose spread over a period of years (or even less) will produce only minor effects on the blood cells. In an extreme case, an acute dose exceeding 600 rems would cause serious illness and in the great majority of instances death could occur within a few weeks. On the other hand, a chronic dose of the same total magnitude accumulated gradually over 20 years might have no observable effect.
12.100 The injury caused by a certain dose (and dose rate) of radiation will depend upon the extent and part of the body that is exposed. One possible reason is that when the exposure is restricted, the unexposed regions may be able to contribute to the recovery of the injured area. But if the whole body is exposed, many organs are affected and recovery is much more difficult.
12.101 Different portions of the body show different sensitivities to ionizing radiations, and there are variations in degree of sensitivity among individuals. In general, the most radiosensitive cells are found in the lymphoid tissue, bone marrow, spleen, organs of reproduction, and gastrointestinal tract. Of intermediate sensitivity are the skin, lungs, and liver, whereas muscle, nerve, and adult bones are the least sensitive.
EFFECTS OF ACUTE RADIATION DOSES
12.102 Before the nuclear bombings of Hiroshima and Nagasaki relatively little was known of the phenomena associated with acute whole-body exposure to ionizing radiation. In Japan, however, a large number of individuals received whole-body doses of radiation ranging from insignificant quantities to amounts which proved fatal. The effects were often complicated by other injuries and shock, so that the symptoms of acute radiation injury could not always be isolated. Because of the great numbers of patients and the lack of facilities after the explosions, it was impossible to make detailed observations and keep accurate records. Nevertheless, certain important conclusions have been drawn from Japanese experience with regard to the effects of nuclear radiation on the human organism.
12.103 Information on this subject has also been gathered from other sources. These include a few laboratory accidents involving a small number of human beings, irradiation used in treating various diseases and malignancies, and extrapolation to man of observations on animals. In addition, detailed knowledge has been obtained from a careful study of over 250 persons in the Marshall Islands, who were accidentally exposed to nuclear radiation from fallout following the test explosion on March 1, 1954 (§ 9.104 et seq.). The exposed individuals included both Marshallese and a small group of American servicemen. The whole-body radiation doses ranged from relatively small values (14 rems), which produced no obvious symptoms, to amounts (175 rems) that caused prompt marked changes in the blood-forming system (§ 12.124).
12.104 No single source of data directly yields the relationship between the physical dose of ionizing radiation and the clinical effect in man. Hence, there is no complete agreement concerning the effect associated with a specific dose or dose range. Attempts in the past have been made to relate particular symptoms to certain narrow ranges of exposure; however, the data are incomplete and associated with many complicating factors that make interpretation difficult. For instance, the observations in Japan were very sketchy until 2 weeks following the exposures, and the people at that time were suffering from malnutrition and pre-existing bacterial and parasitic infections. Consequently, their sickness was often erroneously attributed to the effects of ionizing radiation when such was not necessarily the case. The existing conditions may have been aggravated by the radiation, but to what extent it is impossible to estimate in retrospect.
12.10S In attempting to relate the acute radiation dose to the effect on man, it should be mentioned that reliable information has been obtained for doses up to 200 rems. As the dose increases from 200 to 600 rems, the data from exposed humans decrease rapidly and must be supplemented more and more by extrapolations based on animal studies. Nevertheless, the conclusions drawn can be accepted with a reasonable degree of confidence. Beyond 600 rems, however, observations on man are so sporadic that the relationship between dose and biological effect must be inferred or conjectured almost entirely from observations made on animals exposed to ionizing radiations. Such observations have been made in recent years at extremely high doses.
12.106 Individuals receiving acute whole-body doses of ionizing radiation may show certain signs and symptoms of illness. The time interval to onset of chest symptoms, their severity, and their duration generally depend on the amount of radiation absorbed, although there may be significant variations among individuals. Within any given dose range the effects manifested can be divided conveniently into three time phases: initial, latent, and final.
12.107 During the initial phase, exposed individuals may experience nausea, vomiting, headache, dizziness, and a generalized feeling of illness. The onset time decreases and the severity of these symptoms increases with increasing dose. During the latent phase exposed individuals will experience few, if any, symptoms and most likely will be able to perform useful tasks. The final phase is characterized by illness that requires hospitalization of people receiving the higher doses. In addition to the recurrence of the symptoms noted during the initial phase, skin hemorrhages, diarrhea, and loss of hair may appear, and, at higher doses, seizures and prostration may occur. The final phase is consummated by recovery or death.
12.108 With the foregoing in mind, Table 12.108 is presented as the best available summary of the effects of various whole-body dose ranges of ionizing radiation on human beings. Results of radiobiological studies are generally reported in terms of the (vertical) midline tissue dose in rads. This dose is lower than the dose that would be measured by instruments (and the dose that would be absorbed by tissue) near the surface of the body by a factor that depends upon the energy of the radiation and the size of the individual. The nuclear radiation data presented in Chapters VIII and IX refer to the absorbed dose in tissue at the surface of an individual that is nearest the burst, and thus they also correspond to the expected instrument readings. For consistency, the data in Table 12.108 are the doses (in rems) equivalent to the absorbed doses (in rads) in tissue at the surface of the individual. For gamma rays, these absorbed doses are essentially equal to the exposures in roentgens (§ 8.18). For nuclear weapon radiation, the midline tissue doses for average size adults would be approximately 70 percent of the doses in the table.
Range | 0 to 100 rems Subclinical range |
100 to 1,00 rems Therapeutic range |
Over 1,000 rems Lethal range |
|||
---|---|---|---|---|---|---|
100 to 200 rems | 200 to 600 rems | 600 to 1,000 rems | 1,000 to 5,000 rems | Over 5,000 rems | ||
Clinical surveillance | Therapy effective | Therapy promising | Therapy palliative | |||
Incidence of vomiting | None | 100 rems: infrequent 200 rems: common |
300 rems: 100% | 100% | 100% | |
Initial Phase | ||||||
Onset | — | 3 to 6 hours | ½ to 6 hours | ¼ to ½ hour | 5 to 30 minutes | Almost immediately** |
Duration | — | ≤ 1 day | 1 to 2 days | ≤ 2 days | ≤ 1 day | |
Latent Phase | ||||||
Onset | — | ≤ 1 day | 1 to 2 days | ≤ 2 days | ≤ 1 day* | Almost immediately** |
Duration | — | ≤ 2 weeks | 1 to 4 weeks | 5 to 10 days | 0 to 7 days* | |
Final Phase | ||||||
Onset | — | 10 to 14 days | 1 to 4 weeks | 5 to 10 days | 0 to 10 days | Almost immediately** |
Duration | — | 4 weeks | 1 to 8 weeks | 1 to 4 weeks | 2 to 10 days | |
Leading organ | Hematopoietic tissue | Gastrointestinal tract | Central nervous system | |||
Characteristic signs | None below 50 rems | Moderate leukopenia | Severe leukopenia; purpura; hemorrhage; infection. Epilation above 300 rems. | Diarrhea; fever; disturbance of electrolyte balance. | Convulsions; tremor; ataxia; lethargy. | |
Critical period post-exposure | — | — | 1 to 6 weeks | 2 to 14 days | 1 to 48 hours | |
Therapy | Reassurance | Reassurance; hematologic surveillance. | Blood transfusion; antibiotics. | Consider bone marrow transplantation. | Maintenance of electrolyte balance. | Sedatives |
Prognosis | Excellent | Excellent | Guarded | Guarded | Hopeless | |
Convalescent period | None | Several weeks | 1 to 12 months | Long | — | |
Incidence of death | None | None | 0 to 90% | 90 to 100% | 100% | |
Death occurs within | — | — | 2 to 12 weeks | 1 to 6 weeks | 2 to 14 days | < 1 day to 2 days |
Cause of death | — | — | Hemorrhage; infection | Circulatory collapse | Respiratory failure; brain edema. |
* At the higher doses within this range there may be no latent phase.
** Initial phase merges into final phase, death usually occurring from a few hours to about 2 days; this chronology is possibly interrupted by a very short latent phase.
12.109 As shown in Table 12.108, below 100 rems the response is almost completely subclinical; that is to say, there is no sickness requiring special attention. Changes may, nevertheless, be occurring in the blood, as will be seen later. Between 100 and 1,000 rems is the range in which therapy, i.e., proper medical treatment, will be successful at the lower end and may be successful at the upper end. The earliest symptoms of radiation injury are nausea and vomiting, which may commence within about 15 minutes to 6 hours of exposure, depending on the dose, accompanied by discomfort (malaise), loss of appetite, and fatigue. The most significant, although not immediately obvious effect in the range under consideration, is that on the hematopoietic tissue, i.e., the organs concerned with the formation of blood. An important manifestation of the changes in the functioning of these organs is leukopenia, that is, a decline in the number of leukocytes (white blood cells). Loss of hair (epilation) will be apparent about 2 weeks or so after receipt of a dose exceeding 300 rems.
12.110 Because of the increase in the severity of the radiation injury and the variability in response to treatment in the range from 100 to 1,000 rems, it is convenient to subdivide this range into three subsections, as shown in Table 12.108. For whole-body doses from 100 to 200 rems, hospitalization is generally not required, but above 200 rems admission to a hospital is necessary so that the patient may receive such treatment as may be indicated. Up to 600 rems, there is reasonable confidence in the clinical events and appropriate therapy, but for doses in excess of this amount there is considerable uncertainty and variability in response.
12.111 Beyond 1,000 rems, the prospects of recovery are so poor that therapy may be restricted largely to palliative measures. It is of medical interest, however, to subdivide this lethal range into two parts in which the characteristic major clinical effects are different. Although the dividing line has been somewhat arbitrarily set at 5,000 rems in Table 12.108, human data are so limited that this dose level might well have any value from 2,000 to 6,000 rems. In the range from 1,000 to (roughly) 5,000 rems, pathological changes in the gastrointestinal tract, which are apparent at lower doses, become very marked. Above 5,000 rems, the central nervous system also exhibits the consequences of major injury.
12.112 The superposition of radiation effects upon injuries from other causes may be expected to result in an increase in the number of cases of shock. For example, the combination of sublethal nuclear radiation exposure and moderate thermal burns will produce earlier and more severe shock than would the comparable burns alone. The healing of wounds of all kinds will be retarded because of the susceptibility to secondary infection accompanying radiation injury and for other reasons. In fact, infections, which could normally be dealt with by the body, may prove fatal in such cases.
CHARACTERISTICS OF ACUTE WHOLE-BODY RADIATION INJURY
DOSES OF 25 TO 100 REMS: NO ILLNESS
12.113 Single doses in the range of from 25 to l00 rems over the whole body will produce some changes in the blood (§ 12.124). These changes do not usually occur below this range and are not produced consistently unless the dose is 50 rems or more. Disabling sickness does not occur and exposed individuals should be able to proceed with their usual duties.
DOSES OF 100 TO 200 REMS: SLIGHT OR NO ILLNESS
12.114 A whole-body dose in the range of 100 to 200 rems will result in a certain amount of illness but it will rarely be fatal. Doses of this magnitude were common in Hiroshima and Nagasaki, particularly among persons who were at some distance from the nuclear explosion. Of the 267 individuals accidentally exposed to fallout in the Marshall Islands following the test explosion of March l, 1954, a group of 64 received radiation doses in this range. The exposure of these individuals was not strictly of the acute type, since it extended over a period of some 45 hours. More than half the dose, however, was received within 24 hours and the observed effects were similar to those to be expected from an acute exposure of the same amount.
12.115 The illness from radiation doses in this range does not present a serious problem since most patients will suffer little more than discomfort and fatigue and others may have no symptoms at all. There may be some nausea and vomiting on the first day or so following irradiation, but subsequently there is a latent period of up to 2 weeks or more (§ 12.107). The usual symptoms, such as loss of appetite and malaise, may reappear, but if they do, they are mild. The changes in the character of the blood, which accompany radiation injury, become significant during the latent period and persist for some time. If there are no complications, due to other injuries or infection, there will be recovery in essentially all cases. In general, the more severe the early stages of the radiation sickness, the longer will be the process of recovery. Adequate care and the use of antibiotics, as may be indicated clinically, can greatly expedite complete recovery of the small proportion of more serious cases.
DOSES OF 200 TO 1,000 REMS: SURVIVAL POSSIBLE
12.116 For doses between 200 and 1,000 rems the probability of survival is good at the lower end of the range but poor at the upper end. The initial symptoms are similar to those common in radiation sickness, namely, nausea, vomiting, diarrhea, loss of appetite, and malaise. The larger the dose, the sooner will these symptoms develop, generally during the initial day of the exposure. After the first day or two the symptoms disappear and there may be a latent period of several days to 2 weeks during which the patient feels relatively well, although important changes are occurring in the blood. Subsequently, there is a return of symptoms, including fever, diarrhea, and a steplike rise in temperature which may be due to accompanying infection.
12.117 Commencing about 2 or 3 weeks after exposure, there is a tendency to bleed into various organs, and small hemorrhages under the skin (petechiae) are observed. This tendency may be marked. Particularly common are spontaneous bleeding in the mouth and from the lining of the intestinal tract. There may be blood in the urine due to bleeding in the kidney. The hemorrhagic tendency depends mainly upon depletion of the platelets in the blood, resulting in defects in the blood clotting mechanism (see § 12.129). Loss of hair, which is a prominent con sequence of radiation exposure, also starts after about 2 weeks, i.e., immediately following the latent period, for doses over 300 rems (Fig. 12.117).
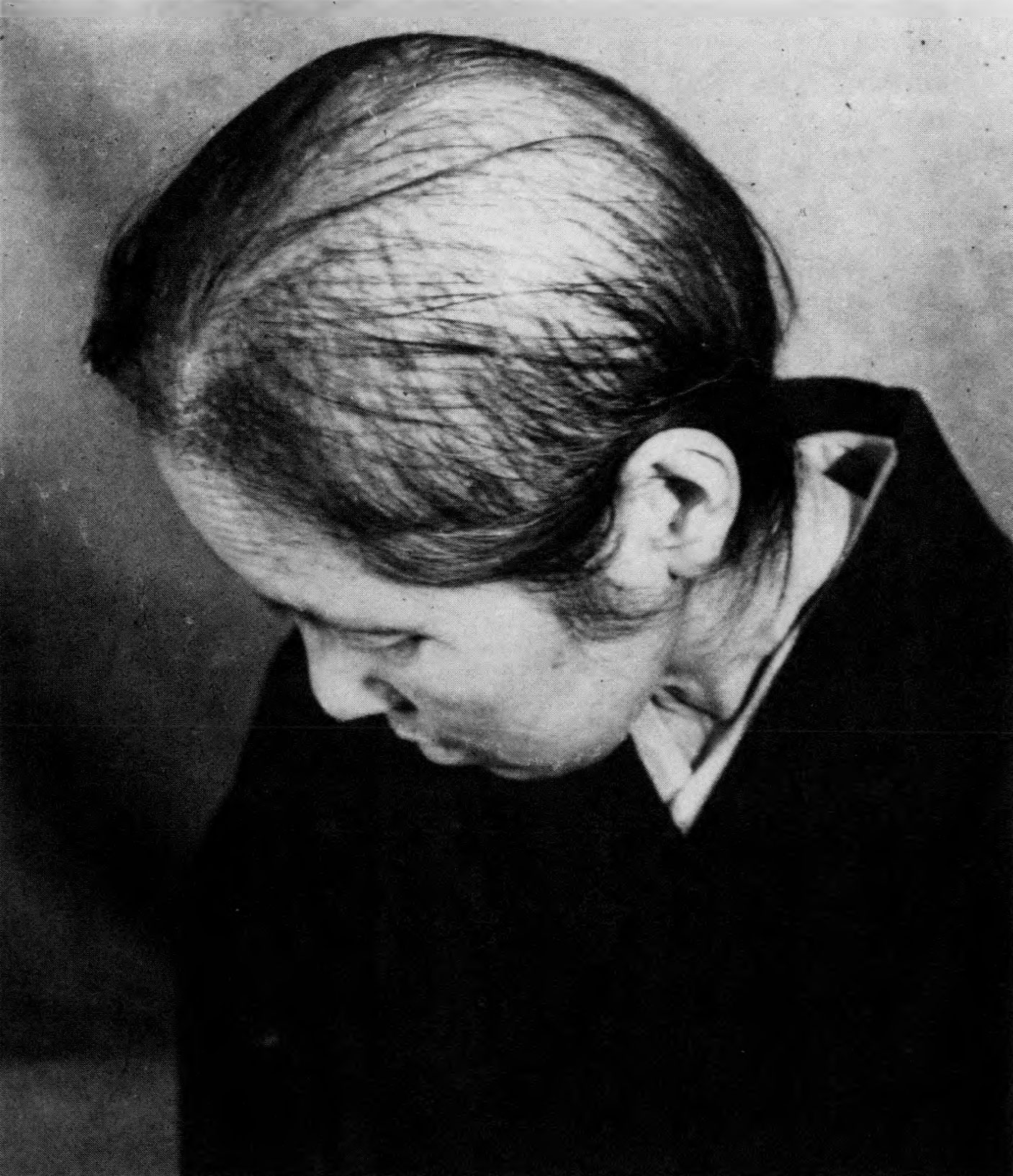
12.118 Susceptibility to infection of wounds, burns, and other lesions, can be a serious complicating factor. This would result to a large degree from loss of the white blood cells, and a marked depression in the body's immunological process. For example, ulceration about the lips may commence after the latent period and spread from the mouth through the entire gastrointestinal tract in the terminal stage of the sickness. The multiplication of bacteria, made possible by the decrease in the white cells of the blood and injury to other immune mechanisms of the body, allows an overwhelming infection to develop.
12.119 Among other effects observed in Japan was a tendency to spontaneous internal bleeding toward the end of the first week. At the same time, swelling and inflammation of the throat was not uncommon. The development of severe radiation illness among the Japanese was accompanied by an increase in the body temperature, which was probably due to secondary infection. Generally there was a step-like rise between the fifth and seventh days, sometimes as early as the third day following exposure, and usually continuing until the day of death.
12.120 In addition to fever, the more serious cases exhibited severe emaciation and delirium, and death occurred within 2 to 8 weeks. Examination after death revealed a decrease in size of and degenerative changes in testes and ovaries. Ulceration of the mucous membrane of the large intestine, which is generally indicative of doses of 1,000 rems or more, was also noted in some cases.
12.121 Those patients in Japan who survived for 3 to 4 months, and did not succumb to tuberculosis, lung diseases, or other complications, gradually re-covered. There was no evidence of permanent loss of hair, and examination of 824 survivors some 3 to 4 years later showed that their blood composition was not significantly different from that of a control group in a city not subjected to nuclear attack.
LARGE DOSE (OVER 1,000 REMS): SURVIVAL IMPROBABLE
12.122 Very large doses of whole body radiation (approximately 5,000 rems or more) result in prompt changes in the central nervous system. The symptoms are hyperexcitability, ataxia (lack of muscular coordination), respiratory distress, and intermittent stupor. There is almost immediate incapacitation for most people, and death is certain in a few hours to a week or so after the acute exposure. If the dose is in the range from 1,000 to roughly 5,000 rems, it is the gastrointestinal system which exhibits the earliest severe clinical effects. There is the usual vomiting and nausea followed, in more or less rapid succession, by prostration, diarrhea, anorexia (lack of appetite and dislike for food), and fever. As observed after the nuclear detonations in Japan, the diarrhea was frequent and severe in character, being watery at first and tending to become bloody later; however, this may have been related to pre existing disease.
12.123 The sooner the foregoing symptoms of radiation injury develop the sooner is death likely to result. Although there may be no pain during the first few days, patients experience malaise, accompanied by marked depression and fatigue. At the lower end of the dose range, the early stages of the severe radiation illness are followed by a latent period of 2 or 3 days (or more), during which the patient appears to be free from symptoms, although profound changes are taking place in the body, especially in the blood-forming tissues. This period, when it occurs, is followed by a recurrence of the early symptoms, often accompanied by delirium or coma, terminating in death usually within a few days to 2 weeks.
EFFECTS OF RADIATION ON BLOOD CONSTITUENTS
12.124 Among the biological consequences of exposure of the whole body to an acute dose of nuclear radiation, perhaps the most striking and characteristic are the changes which take place in the blood and blood-forming organs. Normally, these changes will be detectable only for doses greater than 25 rems. Much information on the hematological response of human beings to nuclear radiation was obtained after the nuclear explosions in Japan and also from observations on victims of laboratory accidents. The situation which developed in the Marshall Islands in March 1, 954, however, provided the opportunity for a very thorough study of the effects of small and moderately large doses of radiation (up to 175 rems) on the blood of human beings (§ 12.103). The descriptions given below, which are in general agreement with the results observed in Japan, are based largely on this study.
12.125 One of the most striking hematological changes associated with radiation injury is in the number of white blood cells. Among these cells are the neutrophils, formed chiefly in the bone marrow, which are concerned with resisting bacterial invasion of the body. During the course of certain types of bacterial infection, the number of neutrophils in the blood increases rapidly to combat the invading organisms. Loss of ability to meet the bacterial invasion, whether due to radiation or any other injury, is a very grave matter, and bacteria which are normally held in check by the neutrophils can then multiply rapidly; the consequences are thus serious. There are several types of white blood cells with different specialized functions, but which have in common the general property of resisting infection or removing toxic products from the body, or both.
12.126 After the body has received a radiation dose in the sublethal range, i.e., about 200 rems or less, the total number of white blood cells may show a transitory increase during the first 2 days or so, and then decrease below normal levels. Subsequently the white count may fluctuate and possibly rise above normal on occasions. During the seventh or eighth weeks, the white cell count becomes stabilized at low levels and a minimum probably occurs at about this time. An upward trend is observed in succeeding weeks but complete recovery may require several months or more.
12.127 The neutrophil count parallels the total white blood cell count, so that the initial increase observed in the latter is apparently due to increased mobilization of neutrophils. Complete return of the number of neutrophils to normal does not occur for several months.
12.128 In contrast to the behavior of the neutrophils, the number of lymphocytes, produced in parts of the lymphatic tissues of the body, e.g., lymph nodes and spleen, shows a sharp drop soon after exposure to radiation. The lymphocyte count continues to remain considerably below normal for several months and recovery may require many months or even years. However, to judge from the observations made in Japan, the lymphocyte count of exposed individuals 3 or 4 years after exposure was not appreciably different from that of unexposed persons.
12.129 A significant hematological change also occurs in the platelets, a constituent of the blood which plays an important role in blood clotting. Unlike the fluctuating total white count, the number of platelets begins to decrease soon after exposure and falls steadily and reaches a minimum at the end of about a month. The decrease in the number of platelets is followed by partial recovery, but a normal count may not be attained for several months or even years after exposure. It is the decrease in the platelet count which partly explains the appearance of hemorrhage and purpura in radiation injury.
12.130 The red blood cell (erythrocyte) count also undergoes a decrease as a result of radiation exposure and hemorrhage, so that symptoms of anemia, e.g., pallor, become apparent. But the change in the number of erythrocytes is much less striking than that in the white blood cells and platelets, especially for radiation doses in the range of 200 to 400 rems. Whereas the response in these cells is rapid, the red cell count shows little or no change for several days. Subsequently, there is a decrease which may continue for 2 or 3 weeks, followed by a gradual increase in individuals who survive.
12.131 As an index of severity of radiation exposure, particularly in the sublethal range, the total white cell or neutrophil counts are of limited usefulness because of the wide fluctuations and the fact that several weeks may elapse before the maximum depression is observed. The lymphocyte count is of more value in this respect, particularly in the low dose range, since depression occurs within a few hours of exposure (§ 12.224). However, a marked decrease in the number of lymphocytes is observed even with low doses and there is relatively little difference with large doses.
12.132 The platelet count, on the other hand, appears to exhibit a regular pattern, with the maximum depression being attained at approximately the same time for various exposures in the sublethal range. Furthermore, in this range, the degree of depression from the normal value is roughly proportional to the estimated whole-body dose. It has been suggested, therefore, that the platelet count might serve as a convenient and relatively simple direct method for determining the severity of radiation injury in the sublethal range. The main disadvantage is that an appreciable decrease in the platelet count is not apparent until some time after exposure.
COMBINED INJURIES
GENERAL CONSIDERATIONS
12.133 Thus far, blast, thermal, and (ionizing) radiation injuries have been considered separately, but combined injuries, from two or more of these causes, would probably be common as a result of a nuclear explosion. Combined injuries might be received almost simultaneously, e.g., from a single detonation without fallout, or separated in time by minutes to days, e.g., from a single detonation followed by fallout or from multiple detonations. Injuries may consist of any combination of blast, thermal, and radiation effects. Furthermore, such injuries may be influenced by other conditions that might be expected during or after a nuclear attack, e.g., malnutrition, poor sanitation, fatigue, and various other environmental factors. Current knowledge concerning combined injuries is derived mainly from studies of the Japanese victims of nuclear bombs and from lab oratory and field tests with a variety of animals.
12.134 The contribution of combined injuries to overall mortality and morbidity in Japan has never been determined adequately, but two general impressions have emerged: the combination of mechanical (blast) and thermal injuries was responsible for the majority of deaths that occurred within the first 48 hours after the attacks, and delayed mortality was higher and complications were more numerous among burned people exposed to ionizing radiation than would have been anticipated in the absence of such radiation. In Hiroshima and Nagasaki, among those who survived for 20 days or more, about 50 percent of the people within 1.25 miles of ground zero received combined injuries, whereas the incidence was roughly 25 percent at distances from 1.25 to 3 miles from ground zero.
12.135 It should be recognized that the incidences of combined injuries in the two Japanese cities apply only to the particular circumstances of the nuclear explosions. The number and types of combined injuries will depend on the yield, height of burst, and the conditions of exposure. Air bursts, unaccompanied by fallout, with yields less than about 10 kilotons, would cause combinations of mechanical, thermal, and initial-radiation injuries. On the other hand, larger yields would be expected to produce a greater proportion of combined burn and mechanical injuries; initial-radiation in juries would be less significant in the surviving population. A nuclear explosion near (above or below) the surface would maximize radiation injuries due to fallout and in a large proportion of the casualties such injuries would be combined with mechanical and thermal effects. People who are outdoors and unshielded would have a greater probability of sustaining initial and/or fallout (residual) radiation injury in combination with flash burns than would those within buildings. In the latter case, burns would be minimized and combinations of mechanical and radiation injuries might be dominant.
12.136 No reliable criteria for incapacitation are known for persons receiving combined injuries. The available data do indicate, however, that individuals suffering such injuries that occur nearly simultaneously are unlikely to become casualties within a few hours, provided the individual injuries would not produce casualties if administered separately. Consequently, it is not unreasonable to make early casualty predictions for a single nuclear detonation on the basis of the most significant injury. If there is a substantial probability of another injury, this could contribute to combined injury and might result in increased casualties at later times.
12.137 The effects of combined injuries may be synergistic, additive, or antagonistic. That is to say, the overall response may be greater than, equal to, or less than, respectively, what would be predicted based on the assumption that the various injuries act independently of one another in producing casualties. Quantitative data from laboratory experiments suggests that in situations where a combined effect has been observed, the interaction of the various forms of injury has resulted in enhanced early as well as delayed mortality, although from the limited data available the latter seems to be the more common.
RADIATION AND THERMAL INJURIES
12.138 Exposure of laboratory animals to external ionizing radiation while subjected to thermal burn has been found to cause a substantial increase in mortality over that expected from the insults received separately. The extent of the increase depends on the radiation dose and the severity of the burn. Severely burned subjects exhibit some anemia and the body is less able to cope with this stress if the immune mechanism and the activity of the bone marrow are depressed by the ionizing radiation. The enhanced mortality from the thermal burns combined with radiation exposure was not observed for doses of 25 rems or less and it is improbable that the synergistic effect would occur unless the dose is large enough to produce at least minimal effect on the immunologic and hematologic systems. Very little information is available on fallout (internal) radiation in combination with thermal or any other form of injury.
MECHANICAL AND RADIATION INJURIES
12.139 Mechanical and radiation injuries can be expected to be frequent, particularly if fallout is present. Studies indicate that there is a delay in wound healing with doses in excess of 300 rems, and that wounds in irradiated subjects are considerably more serious if treatment is delayed for more than 24 hours. In addition, missile and impact injuries that result in disruption of the skin and damage to the soft tissues provide a portal of entry for infection, and thus may be extremely hazardous to irradiated people. Injuries that are associated with significant blood loss would be more serious in those who have received a radiation dose large enough to interfere with normal blood clotting mechanisms.
12.140 One week after exposure to an external radiation dose which would by itself have resulted in 45 percent mortality within 30 days, animals (rats) were subjected to a blast overpressure which would normally produce 5 per cent early lethality. As a result, early lethality associated with blast-induced hemorrhage and lung injury was increased four fold and the delayed mortality was almost double that expected from the radiation alone. In these tests, ionizing radiation and blast were clearly synergistic in causing both early and delayed mortality.
THERMAL AND MECHANICAL INJURIES
12.141 Burns and mechanical injuries in combination are often encountered in victims of conventional explosions. Increased numbers of delayed complications, shorter times-to-death, and enhanced mortality rate are frequent occurrences. However, few quantitative data are available on this form of combined injury.
LATE EFFECTS OF IONIZING RADIATION
INTRODUCTION
12.142 There are a number of consequences of nuclear radiation which may not appear for some years after exposure. Among them, apart from genetic effects, are the formation of cataracts, nonspecific life shortening, leukemia, other forms of malignant disease, and retarded development of children in utero at the time of the exposure. Information concerning these late effects has been obtained from continued studies of various types, including those in Japan made chiefly under the direction of the Atomic Bomb Casualty Commission.10
12.143 The effects which occur later in life, like the acute reactions observed within a few weeks or months after irradiation, arise from changes in cells and tissues at the time of exposure. If an exposed individual survives the acute reaction, cell replacement may be complete, but the cells may not necessarily be quite normal; however, the causes for the late effects are largely unknown although many theories have been proposed.
CATARACTS
12.144 The term “cataract” is commonly used to describe any detectable change in the normal transparency of the lens of the eye. Cataracts may range from small lesions, which cause only minor impairment of vision, to extensive opacification that results in total blindness. The vast majority of natural cataracts in man are of the senile form of arthritis known as ankylosing spondylitis. Three main types of leukemia are induced by radiation, namely, acute and chronic granulocytic and acute lymphocytic forms; the occurrence of chronic lymphocytic leukemia is not significantly increased by radiation. The development of leukemia as a result of an overexposure to radiation is associated with a latent period varying from one to 20 years or more. The disease is generally fatal, no matter what its cause.
12.148 The first evidence of an increased incidence of leukemia among the survivors of the Hiroshima and Nagasaki explosions appeared for 1947. The occurrence of the disease reached a peak in 1951 and 1952 and it has been declining since then. By the end of 1966, the frequency of acute granulocytic anemia was approaching the normal value for Japan. Children who were exposed to radiation when they were less than 10 years old were roughly twice as susceptible to leukemia as older individuals. One case of acute granulocytic leukemia was discovered in 1972 among the 53 inhabitants of Rongelap Atoll in the Marshall Islands who had received an estimated whole-body dose of 175 rems of gamma radiation from fallout in 1954 (§ 12.175 et seq.). The individual, a young man, had been a year old at the time of exposure.
12.149 The occurrence of leukemia, for a given estimated absorbed dose (in rads), appeared to be greater in Hiroshima than in Nagasaki. Later studies revealed that the Hiroshima (gun type, uranium-235) bomb emitted a larger proportion of neutrons, relative to gamma rays, than did the Nagasaki (implosion type, plutonium-239) device. By attributing an RBE of about 5 for the induction of leukemia by fast neutrons, the incidences (per rem) in the two cities were in general agreement. The evidence from Japan, and from other sources, is that the probability of the occurrence of leukemia is roughly proportional to the whole-body dose, and there is no indication of a threshold value. About 90 percent of the cases of leukemia among the survivors in Hiroshima and Nagasaki received doses of more than 200 rems, but not all the people who received such large doses developed the disease. An approximate estimate suggests that there were about 20 instances of leukemia per rem per million population exposed at age 10 years or more and roughly twice this number for younger individuals.
OTHER TYPES OF CANCER
12.150 It has been established from the mortality statistics of radiologists and of some of the spondylotic patients mentioned in § 12.147, from other exposures to radiation for various medical purposes, and from experiments with animals that large doses of radiation can increase the frequency of various types of cancer, in addition to leukemia. The same effect has been observed among the survivors of the nuclear attacks on Japan. For example, after a latent period of about 10 years, a significant increase was observed in the incidence of thyroid cancer among individuals who were within about half a mile from ground zero and consequently received large doses of ionizing radiation. Delayed thyroid abnormalities have also been found among the inhabitants of the Marshall Islands whose glands were subjected to internal exposure from radioiodine in fallout, but only a small proportion were malignant (§ 12.181). The frequency of thyroid cancer induced by radiation is estimated to be roughly 10 per rem per million of exposed adults, but substantially more for children. Provided it is detected in time, however, thyroid cancer is rarely fatal in children and only in about 10 percent of adults.
12.151 A statistical study of mortality data, obtained from 1950 through 1970, of a large number of people who were in Hiroshima and Nagasaki at the times of the nuclear explosions shows an increased frequency of various other types of cancer. The most important sites appear to be the lung, the gastrointestinal system (other than the stomach), and the female breast. Although they are relatively rare, salivary gland tumors have been found to be more common among the Japanese exposed to radiation than in the unexposed population. In a group of 109,000 survivors who have been studied about 5,700 received whole-body doses of 100 rems or more. Among these, 690 were over 50 years of age at the time of exposure and during the period from 1960 to 1970 there were 47 deaths from cancer, other than leukemia, whereas about 30 would have been expected. Of the 820 children who were under 10 years of age when exposed, there were six such deaths, compared with 0.75 expected. Thus, although the actual increase in fatal cancers was smaller among those exposed at an early age, the relative increase, i.e., actual/expected, was much greater than in older persons.
LIFE SHORTENING
12.152 Laboratory studies with animals have indicated that shortening of the life span, apart from the effects of leukemia and other forms of malignant disease, can sometimes (but not always) result from partial or whole-body exposure to radiation. Such shortening may be the result of a number of factors, including decreased immunity to infection, damage to connective tissues, and possibly premature aging. The life shortening in a given animal, for a specific radiation dose, apparently depends on such factors as genetic constitution and on the age and physical condition at the time of the exposure.
12.153 It has been reported that for radiologists who received fairly large chronic doses of radiation in the course of their work, before adequate protective measures were instituted, the average age at death was about five years less than for other physicians. Part of the increase in death rate was due to leukemia and other forms of cancer, but after allowing for these and other specific effects of radiation, there were indications that ionizing radiations caused nonspecific life shortening. However, an examination of deaths occurring from 1950 through 1970 of survivors of the nuclear attacks on Japan suggests that, apart from various forms of cancer, there is little evidence that radiation accelerated aging.
RETARDED DEVELOPMENT OF CHILDREN
12.154 Among the mothers who were pregnant at the time of the nuclear explosions in Japan, and who received sufficiently large doses to show the usual acute radiation symptoms, there was a marked increase over normal in the number of stillbirths and in the deaths of infants within a year of birth. The increase in mortality was significant only when the mothers had been exposed during the last three months of pregnancy. Among the surviving children there was a slight increase in frequency of mental retardation and head circumferences were smaller than normal. These effects were most marked when the radiation exposure occurred within the first three or four months of pregnancy. Most of the mothers of the children referred to above were so close to ground zero that they must have received more than 200 rems of ionizing radiation. Maldevelopment of the teeth, attributed to injury to the roots, was also noted in many of the children. Children who were conceived after the nuclear attacks, even by irradiated parents, appear for the most part to be normal. The fear expressed at one time that there would be a sharp increase in the occurrence of abnormalities has not been substantiated.
EFFECTS OF EARLY FALLOUT
EXTERNAL HAZARD: BETA BURNS
12.155 In most circumstances, the whole-body dose from the gamma rays emitted by the early fallout will represent the major external hazard from the delayed nuclear radiation. The biological effects are then similar to those from equal acute doses of radiation (§ 12.102 et seq.). In addition, injury can arise in two general ways from external sources of beta particles. If the beta-particle emitters, e.g., fission products, come into actual contact with the skin and remain for an appreciable time, a form of radiation injury, sometimes referred to as “beta burn,” will result. In addition, in an area of extensive early fall out, the whole surface of the body may be exposed to beta particles coming from many directions. It is true that clothing will attenuate this radiation to a considerable extent; nevertheless, the whole body could receive a large dose from beta particles which might be significant.
12.156 Information concerning the development and healing of beta burns has been obtained from observations of the Marshall Islanders who were exposed to fallout in March 1954 (§ 12.103). Within about 5 hours of the burst, radioactive material commenced to fall on some of the islands. Although the fallout was observed as a white powder, consisting largely of particles of lime (calcium oxide) resulting from the decomposition of coral (calcium carbonate) by heat, the island inhabitants did not realize its significance. Because the weather was hot and damp, the Marshallese remained outdoors; their bodies were moist and they wore relatively little clothing. As a result, appreciable amounts of fission products fell upon the hair and skin and remained there for a considerable time. Moreover, since the islanders, as a rule, did not wear shoes, their bare feet were continually subjected to contamination from fallout on the ground.
12.157 During the first 24 to 48 hours, a number of individuals in the more highly contaminated groups experienced itching and a burning sensation of the skin. These symptoms were less marked among those who were less contaminated with early fallout. Within a day or two all skin symptoms subsided and disappeared, but after the lapse of about 2 to 3 weeks, epilation and skin lesions were apparent on the areas of the body that had been contaminated by fallout particles. There was apparently no erythema, as might have been expected, but this may have been obscured by the natural coloration of the skin.
12.158 The first evidence of skin damage was increased pigmentation, in the form of dark colored patches and raised areas (macules, papules, and raised plaques). These lesions developed on the exposed parts of the body not protected by clothing, and occurred usually in the following order: scalp (with epilation), neck, shoulders, depressions in the forearm, feet, limbs, and trunk. Epilation and lesions of the scalp, neck, and foot were most frequently observed (Figs. 12.158a and b).
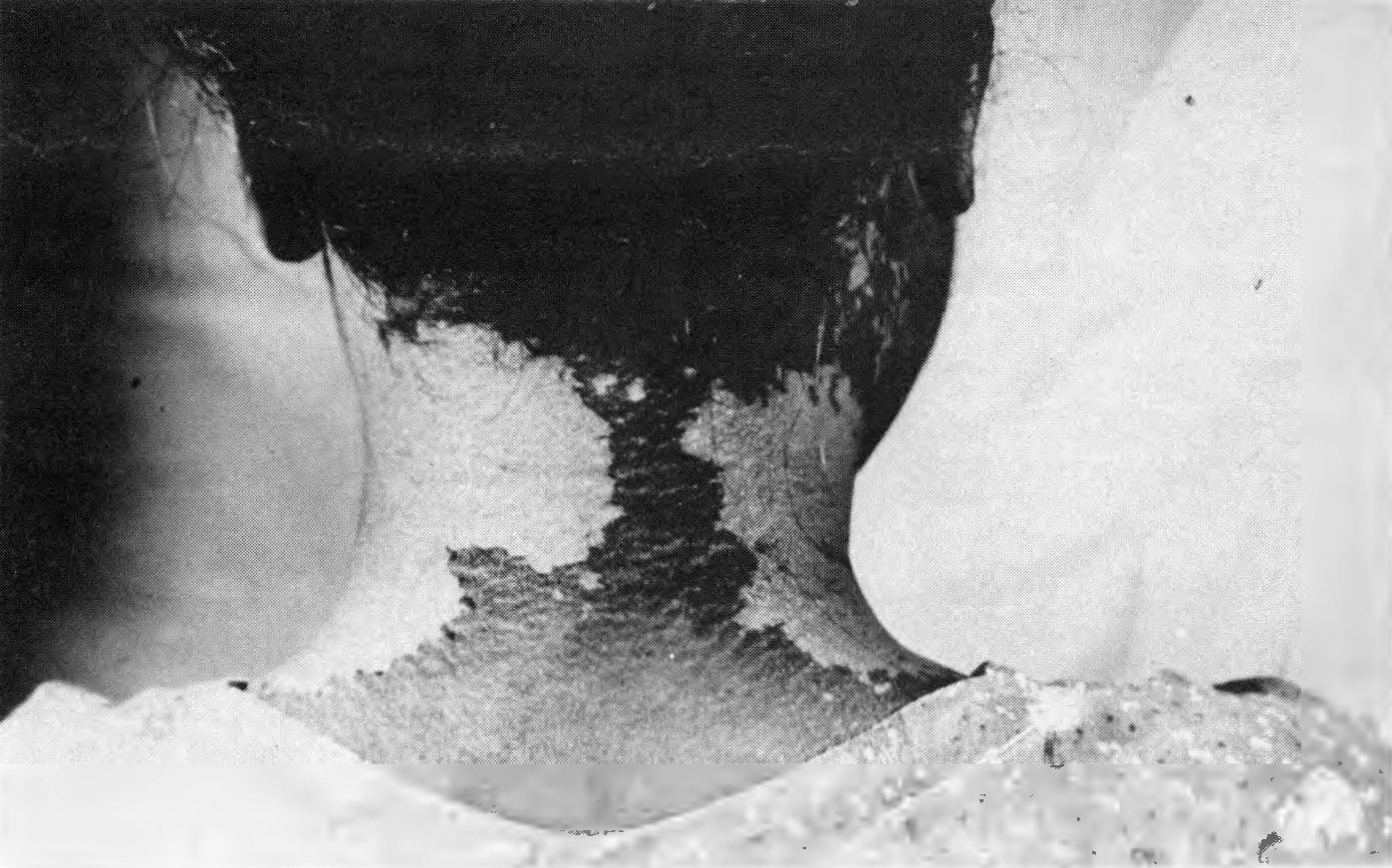
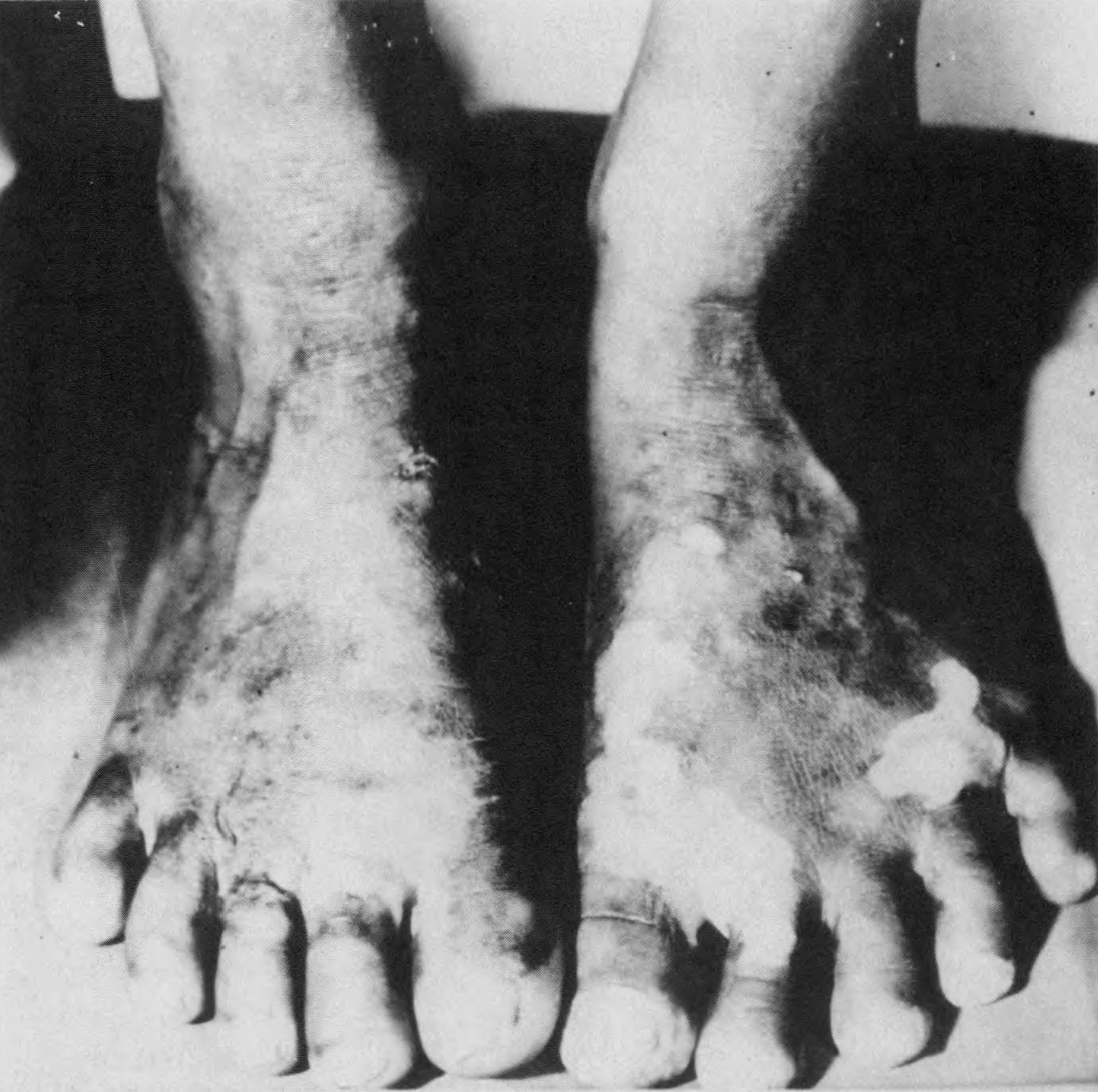
12.159 In addition, a bluish-brown pigmentation of the fingernails was very common among the Marshallese and also among American negroes who were in a group of servicemen stationed on Rongerik Atoll (Fig. 9.105). The phenomenon appears to be a radiation response peculiar to the dark-skinned races, since it was not apparent in any of the white Americans who were exposed at the same time. The nail pigmentation occurred in a number of individuals who did not have skin lesions. It is probable that this was caused by gamma rays, rather than by beta particles, as the same effect has been observed in dark-skinned patients undergoing X-ray treatment in clinical practice.
12.160 Most of the lesions were superficial without blistering. Microscopic examination at 3 to 6 weeks showed that the damage was most marked in the outer layers of the skin (epidermis), whereas damage to the deeper tissue was much less severe. This is consistent with the short range of beta particles in animal tissue. After formation of dry scab, the lesions healed rapidly leaving a central depigmented area, surrounded by an irregular zone of increased pigmentation. Normal pigmentation gradually spread outward in the course of a few weeks.
12.161 Individuals who had been more highly contaminated developed deeper lesions, usually on the feet or neck, accompanied by mild burning, itching, and pain. These lesions were wet, weeping, and ulcerated, becoming covered by a hard, dry scab; however, the majority healed readily with the regular treatment generally employed for other skin lesions not connected with radiation. Abnormal pigmentation effects persisted for some time, and in several cases about a year elapsed before the normal (darkish) skin coloration was restored (Figs. 12.161a and b).
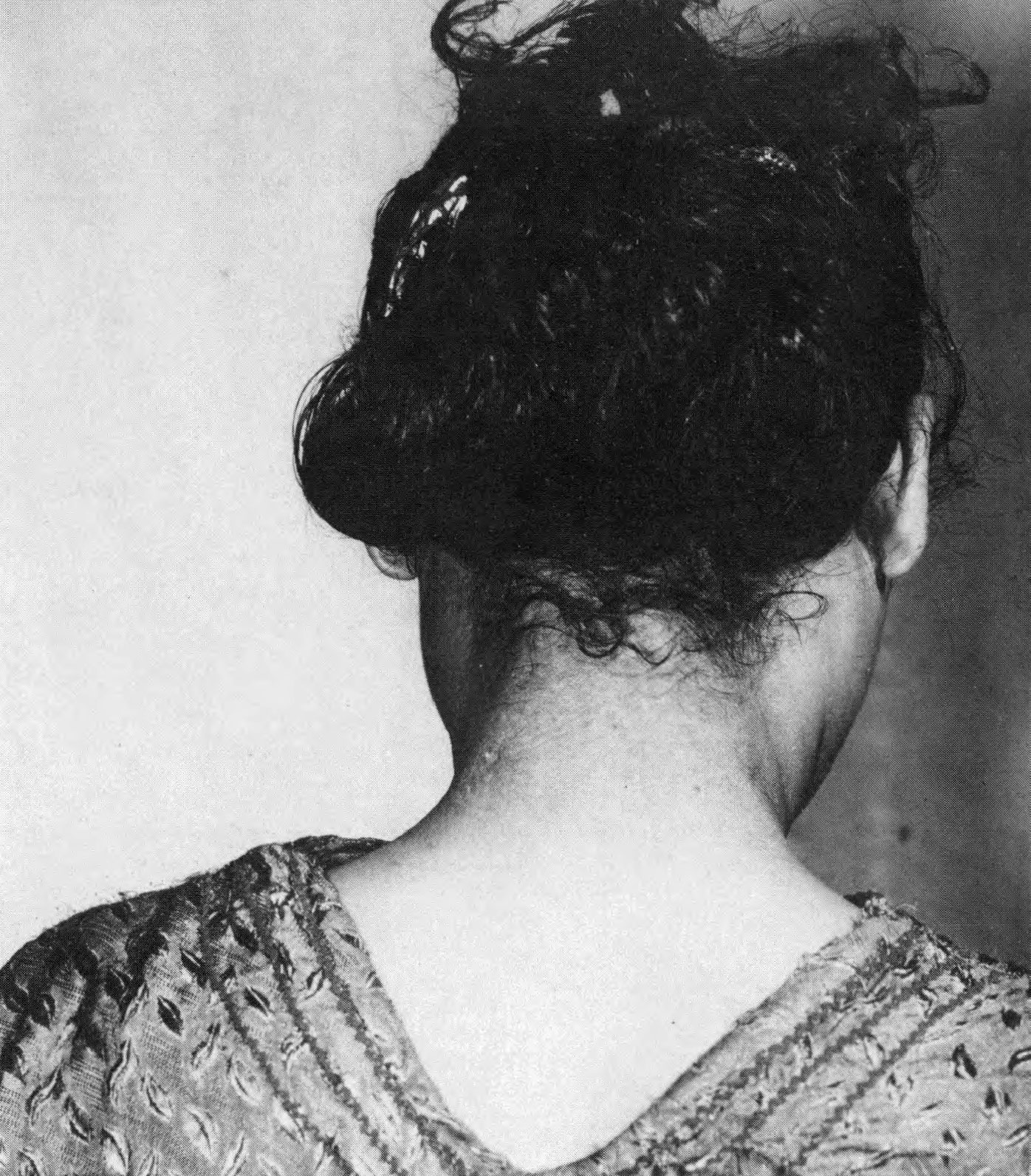
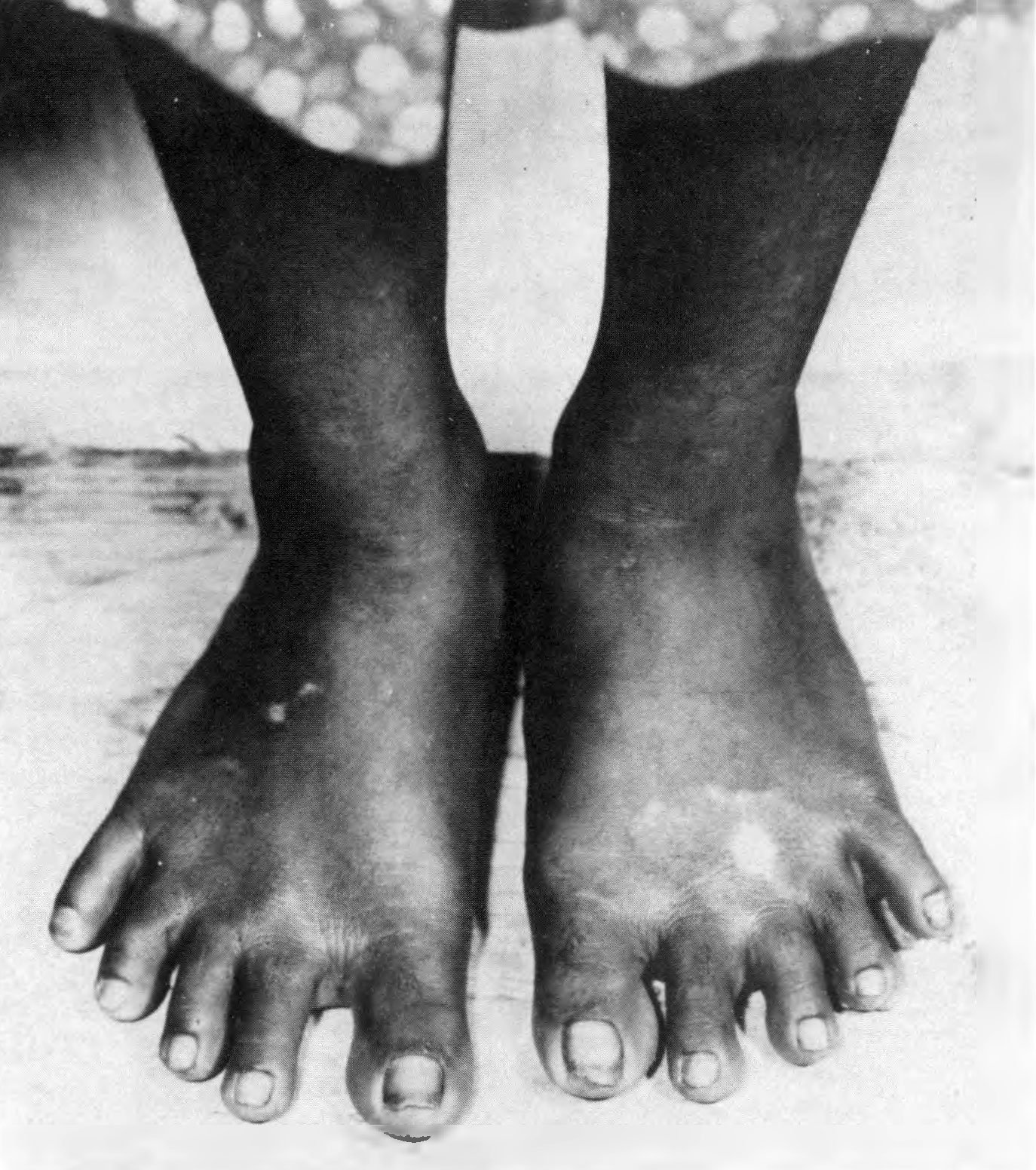
12.162 Regrowth of hair, of the usual color (in contrast to the skin pigmentation) and texture, began about 9 weeks after contamination by fallout and was complete in 6 months. By the at the same time, nail discoloration had grown out in all but a few individuals. Seven years later, there were only 10 cases which continued to show any effects of beta burns, and there was no evidence of malignant changes.
INTERNAL HAZARD
12.163 Wherever fallout occurs there is a chance that radioactive material will enter the body through the digestive tract (due to the consumption of food and water contaminated with fission products), through the lungs (by breathing air containing fallout particles), or through wounds or abrasions. Even a very small quantity of radioactive material if retained in the body can produce considerable injury. Radiation exposure of various organs and tissues from internal sources is continuous, subject only to depletion of the quantity of active material in the body as a result of physical (radioactive decay) and biological (elimination) processes. Furthermore, internal sources of alpha emitters, e.g., plutonium, or of beta particles, or soft (low-energy) gamma ray emitters, can deposit their entire energy within a small, possibly sensitive, volume of body tissue, thus causing considerable damage. Even if the radioisotope remains in the body for a fairly short time and causes no observable early injury, it may contribute to damage that does not become apparent for some time (§ 12.142 et seq.).
12.164 The situation with regard to internal exposure is sometimes aggravated by the fact that certain chemical elements tend to concentrate in specific organs or tissues, some of which are highly sensitive to ionizing radiation. The fate of a given radioactive element which has entered the bloodstream will depend upon its chemical nature. Radioisotopes of an element which is a normal constituent of the body will follow the same metabolic processes as the naturally occurring, inactive (stable) isotopes of the same element. This is the case, for example, with iodine isotopes, all of which-radioactive and stable tend to concentrate in the thyroid gland.
12.165 An element not usually found in the body, except perhaps in minute traces, will behave like one with similar chemical properties that is normally present. Thus, among the fission products, strontium and barium, which are similar chemically to calcium, would be largely deposited in the calcifying tissue of bone. The radioisotopes of the rare earth elements, e.g., cerium, which constitute a considerable proportion of the fission products, and plutonium, which may be present to some extent in the fallout, are also “bone-seekers.” Since they are not chemical analogues of calcium, how ever, they are deposited to a smaller extent and in other parts of the bone than are strontium and barium. Bone-seeking radioisotopes are potentially hazardous for two reasons in particular; first, the radiations can damage the bone marrow and thus affect the whole body by decreasing blood-cell formation (§ 12.226), and second, the deposition of alpha- or beta-particle energy in a small volume can cause serious bone damage, including cancer (§ 12.173).
12.166 The extent to which early fallout contamination can enter the bloodstream as a result of ingestion, inhalation, or a wound is strongly influenced by the physical properties, e.g., size distribution, density, and surface area, of the particles, and by their solubility in the body fluids. Whether the material is subsequently deposited in some specific tissue or not will be determined by the chemical properties of the elements present, as indicated previously. Elements which do not tend to concentrate in a particular part of the body are eliminated fairly rapidly by natural processes.
12.167 The amount of radioactive material absorbed from early fallout by inhalation appears to be relatively small because the nose can filter out almost all particles over IO micrometers (see § 2.27 footnote) in diameter, and about 95 percent of those exceed 5 micrometers. Although particles of a wide range of sizes will be present, most of the particles descending in the fallout during the critical period of highest activity, e.g., within 24 hours of the ex plosion, will be the larger ones (§ 9.50), more than 10 micrometers in diameter. Consequently, only a small proportion of the early fallout particles present in the air will succeed in reaching the lungs. Furthermore, the optimum size for deposition in the alveolar (air) cells of the lungs is as small as 1 to 2 micrometers.
12.168 Since many of the contaminated particles are relatively insoluble, the probability is low that inhaled fission products and other weapon residues present in the early fallout will reach the bloodstream from the lungs. After deposition in the alveolar spaces of the lungs, particles of low solubility in the body fluids may be retained in these spaces for long periods until they are eventually dissolved or are removed by mechanical means, e.g., by cellular or lymphatic transport or in mucus. Particles leaving the lungs by way of the lymphatic system tend to accumulate principally in the tracheobronchial lymph nodes thereby leading to an intense, localized radiation dose.
12.169 Following ingestion or clearance of the upper respiratory tract after inhalation, the extent of absorption of fission products and other radioactive materials through the intestine is largely dependent upon the solubility of the particles. In the early fallout, the fission products as well as uranium and plutonium are chiefly present as oxides, many of which do not dissolve to any great extent in body fluids. The oxides of strontium and barium, however, are soluble, so that these elements enter the blood stream more readily and find their way into the bones.11 The element iodine is also chiefly present in a soluble form and so it soon enters the blood and is concentrated in the thyroid gland.
12.170 The length of time a particular nuclide remains in the body depends on its radioactive half-life (§ 1.63), which determines the rate of removal by natural decay, and on its “biological half-life,” i.e., the time for the amount in the body to decrease to half of its initial value solely as a result of elimination by biological processes. The combination of radioactive and biological half-lives leads to the “effective half-life” as a measure of the net rate of loss of the radionuclide from the body by both decay and biological elimination. The retention pattern of a given element in the body represents the summation of the retentions in individual tissues. In those cases where practically all the body burden is in one tissue (or organ), e.g., iodine in the thyroid gland, the effective half-life is essentially that for this tissue (or organ). A major consideration in assessing the internal hazard from a given radionuclide is the total radiation dose (in rems) delivered while it is in the body (or a critical organ). The main factors in this respect are the effective half-life, which determines the time the nuclide is present in the body (or organ), the total quantity in the body (or organ), and the nature and energy of the radiation emitted. The importance of these factors in various circumstances will become apparent in due course.
12.171 The biological half-life of the element iodine, which is essentially that in the thyroid gland, has an average value of about 80 days, although it actually varies from a few days in some people to several years in others. A number of radioactive isotopes of iodine are present among the fission products, but most have moderate or short radioactive half-lives. The effective half lives, which are related to the times the various isotopes are effective in the body (thyroid), are then determined mainly by the radioactive half-lives, rather than by the longer biological half-life. The heavier isotopes, iodine-132, -133, -134, etc., all of which have radioactive half-lives of less than a day, thus have short effective half-lives; consequently, they constitute a hazard only if delivered in sufficient amounts to the thyroid via the bloodstream. The injury that might be caused by these isotopes is then largely dependent on the quantities that reach the thyroid gland within a short time. On the other hand, the common fission product iodine-131 , with a half-life of about 8 days, has a longer effective half-life and can represent a hazard in smaller amounts because it remains active in the thyroid for a longer time.
12.172 In addition to radioiodine, the important potentially hazardous fission products, assuming sufficient amounts get into the body, fall into two groups. The first, and more significant, contains strontium-89, strontium-90, cesium-137, and barium-140, whereas the second consists of a group of rare earth and related elements, particularly cerium-144 and the chemically similar yttrium-91.
12.173 Another potentially hazardous element, which may be present to some extent in the early fallout, is plutonium, in the form of the alpha-particle emitting isotope plutonium-239. This isotope has a long radioactive half-life (24,000 years) as well as a long biological half-life in the skeleton (about 100 years) and the liver (about 40 years). As with any airborne particulate matter, a fraction of the inhaled fallout particles contaminated with plutonium will be deposited in the alveolar spaces of the lungs. If the particles are relatively insoluble, they can be retained in the lungs for long periods with gradual removal by mechanical means or by slow absorption in the blood. With the more soluble particles, residence time in the lungs will be shorter and absorption into the bloodstream will occur more rapidly. Plutonium that enters the bloodstream tends to be deposited in the liver and on certain surfaces of the bone; the amount of plutonium present and its activity decrease at a very slow rate because of the long radioactive and biological half-lives. The continuous exposure for many years of a limited region of the body, e.g., lung, liver, or bone surface, to the short-range but high-energy alpha particles from plutonium can cause serious injury. Thus, the injection of sufficient amounts of soluble plutonium into some animals has been found to cause bone malignancies whereas inhalation of plutonium dioxide particles may result in the formation of lung tumors.
12.174 Despite the large amounts of radioactive material which may pass through the kidneys in the process of elimination, these organs ordinarily are not greatly affected by radiation. By contrast, uranium can cause damage to the kidneys, but as a chemical poison rather than because of its radioactivity. However, the quantity of uranium compounds found in the fallout that must be ingested in order to be potentially poisonous are so large that it is not considered to be of primary concern compared with other constituents of nuclear weapon debris.
MARSHALLESE EXPERIENCE
12.175 Early fallout accompanying the nuclear air bursts over Japan was insignificant and was not monitored. Consequently, no information was available concerning the potentialities of fission products and other weapon residues as internal sources of radiation. Following the incident in the Marshall Islands in March 1954, however, data of great interest were obtained. Because they were not aware of the significance of the fallout, many of the inhabitants ate contaminated food and drank contaminated water from open containers for periods up to 2 days before they were evacuated from the islands.
12.176 Internal deposition of fission products resulted mainly from ingestion rather than inhalation for, in addition to the reasons given above, the radioactive particles in the air settled out fairly rapidly, but contaminated food, water, and utensils were used all the time. The belief that ingestion was the chief source of internal contamination was supported by the observations on chickens and pigs made soon after the explosion. The gastrointestinal tract, its contents, and the liver were found to be much more contaminated than lung tissue.
12.177 From radiochemical analysis of the urine of the Marshallese subjected to the early fallout, it was possible to estimate the body burdens, i.e., the amounts deposited in the tissues, of various isotopes. It was found that iodine-131 made the major contribution to the activity at the beginning, but it soon disappeared because of its relatively short radioactive half-life (8 days). Somewhat the same was true for barium-140 (12.8 days half-life), but the activity levels of the strontium isotopes were more persistent. Not only do these isotopes have longer radioactive half lives, but the biological half-life of the element is also relatively long.
12.178 No elements other than iodine, strontium, barium, and the rare earth group were found to be retained in appreciable amounts in the body. Essentially all other fission products and weapon residue activities were rapidly eliminated, because of either the short effective half-lives of the radionuclides, the sparing solubility of the oxides, or the relatively large size of the fallout particles.
12.179 The body burden of radioactive material among the more highly contaminated inhabitants of the Marshall Islands was never very large and it decreased fairly rapidly in the course of 2 or 3 months. The activity of the strontium isotopes fell off somewhat more slowly than that of the other radioisotopes, because of the longer radioactive half-lives and greater retention in the bone. Nevertheless, even strontium could not be regarded as a dangerous source of internal radiation in the cases studied. At 6 months after the explosion, the urine of most individuals contained only barely detectable quantities of radioactive material.
12.180 In spite of the fact that the Marshallese people lived approximately 2 days under conditions where maximum probability of contamination of food and water supplies existed and that they took few steps to protect themselves, the amount of internally deposited radioactivity from early fallout was small. There seems to be little doubt, therefore, that, at least as far as short term effects are concerned, the radiation injury by early fallout due to internal sources can be minor in comparison with that due to the external radiation. However, delayed effects of internal radiation exposure, including one case of leukemia (§ 12.148), became apparent several years after the explosion.
12.181 Until 1963, no thyroid abnormalities had been detected among the inhabitants of the Marshall Islands that could be attributed to the fallout. In that year, one was found among the people of Rongelap Atoll, but by 1966 there were 18 cases; the total number increased to 22 by 1969 and to 28 by 1974. Of the Rongelap people who were exposed, 64 (plus one in utero) received external doses of about 175 rems; 18 others (plus one in utero), who were on the neighboring Alinginae Atoll (cf. Fig. 9.105) at the time of the nuclear test, received about 69 rems. The thyroid doses from radioiodine were much larger, especially in children under 16 years of age. In 1974, there were 22 individuals with thyroid lesions among the more highly exposed group and six among the others. In the former group there were three malignancies and two cases of atrophied thyroids (hypothyroidism); there were no definite malignancies in the latter group although there was one doubtful case. All other thyroid abnormalities were benign nodules.
12.182 Most of the lesions occurred in children who were less than 10 years old at the time of the explosion in 1954. Of a total of 19 such children who were on Rongelap, 17 developed abnormalities, including one malignancy and two cases of hypothyroidism. The radiation doses from radioiodine isotopes that had been concentrated in the thyroids of these children were estimated to be from 810 to 1,150 rems. In 1974, a lesion was observed in one of the individuals who had been exposed in utero; the thyroid dose was uncertain but it must have been at least 175 rems. The six children in the less highly exposed group who were on Alinginae received estimated thyroid doses of 275 to 450 rems; by 1974, lesions were observed in two cases with one doubtful malignancy.
12.183 For purposes of comparison, studies were made on 194 people who normally lived on Rongelap Atoll but who were away on other islands and were not exposed to the fallout. There were nine thyroid abnormalities (non malignant), including one in 61 children who were less than 10 years old in 1954. An examination was also made of 157 inhabitants of Utirik Atoll who had received external doses of 14 rems from the fallout. The 58 children less than 10 years old at the time of the explosion received thyroid doses from radioiodines estimated to be 60 to 95 rems, but by 1974 no abnormalities had been observed. Six people in the older group of 99 were found to have thyroid lesions, one of which was malignant; the estimated thyroid doses were in the range of 27 to 60 rems.
LONG-TERM HAZARD FROM DELAYED FALLOUT12
CESIUM-137
12.184 Of the fission products which present a potential long-term hazard from either the atmospheric testing of nuclear weapons in peacetime or their use in warfare, the most important are probably the radioactive isotopes cesium-137 and strontium-90. Since both of these isotopes are fairly abundant among the fission products and have relatively long half-lives, they will constitute a large percentage of any delayed fallout. The process of fractionation will tend to increase the proportions of strontium and cesium still further (§ 9.08). Of course, the activity level due to these isotopes at late times in the early fallout pattern in the area close to a surface or subsurface burst will be considerably larger than in the delayed fallout from a given explosion. However, the special interest in the delayed fallout arises from the fact that it may occur in significant amounts in many parts of the globe remote from the point of the nuclear detonation, as explained in Chapter IX, as well as in close by areas.
12.185 Cesium-137 has a radioactive half-life of 30 years and is of particular interest in fallout that is more than a year old because it is the principal constituent whose radioactive decay is accompanied by the emission of gamma rays. The chemical and biochemical properties of cesium resemble those of potassium. The compounds of these elements are generally more soluble than the corresponding compounds of strontium and calcium and the details of the transfer of these two pairs of elements from the soil to the human body are quite different. The element cesium is relatively rare in nature and the body normally contains only small traces. Because of the presence of cesium-137 in the delayed fallout, studies have been made of the behavior of this isotope in various biological systems and of the levels of uptake and retention in man. Regardless of its mode of entry inhalation, ingestion, or wounds-cesium is soon distributed fairly uniformly throughout the body. A preferential deposition in muscle results in concentrations that are somewhat higher than in the body as a whole, whereas in some other tissues, e.g., the lungs and skeleton, the concentrations are lower than the body average.
12.186 From the studies referred to above, the biological half-life of cesium in human adults has been reported as ranging from 50 to 200 days. Factors contributing to this spread of values include diet, age, sex, race, and body weight. Because of the fairly uniform distribution of cesium, the entire body would be irradiated by both beta particles and gamma rays emitted as the cesium-137 decays. However, since the biological half-life of cesium is relatively short, compared with strontium, and it does not tend to concentrate significantly in any organ or tissue, the residual cesium-137 in a given amount of delayed fallout is much less of a biological hazard than is the strontium-90.
12.187 The amount of internal exposure to cesium-137 is determined by the quantity of this isotope in food. If the major mechanism for its incorporation into the diet is through the root systems of plants, then the dose will be more or less proportional to the total amount of cesium-137 accumulated on the ground. On the other hand, if this isotope enters the diet mainly through material deposited directly on the leaves of plants, the internal dose will be more nearly proportional to the rate of descent of delayed fallout. It has been calculated that if the former mechanism prevails, the internal 30-year does to the gonads, which is of interest in connection with possible genetic effects (§ 12.201 et seq.), would be much higher than if the alternative mechanism were of major importance. The best data presently available on cesium-137 levels in food suggest that, up to the present time, the fallout rate has been the dominant factor; but in the future a larger proportion of the cesium may get into food via the soil, provided no considerable amounts of cesium-137 are added to the atmosphere.
STRONTIUM-90
12.188 Strontium-90, because of its relatively long radioactive half-life of 27.7 years and its appreciable yield in the fission process, accounts for a considerable fraction of the total activity of fission products which are several years old. Strontium is chemically similar to calcium, an element essential to both plant and animal life; an adult human being, for example, contains over 2 pounds of calcium, mainly in bone. However, the relationship between strontium and calcium is not a simple one as will be seen in subsequent sections and, because of its complex metabolism in the body, the behavior of strontium-90 cannot be stated in terms of a single effective half-life (§ 12.170). 13
12.189 The probability of serious pathological change in the body of a particular individual, due to the effects of radioisotopes deposited internally, depends upon the amount deposited, the energy of the radiations emitted, and the length of time the source remains in the body. Strontium-90 and its daughter, yttrium-90, emit beta particles which can cause serious localized damage following their deposition and long-term retention in the skeleton.14 Tests with animals indicate that the pathological effects resulting from sufficient quantities of inhaled, ingested, or injected strontium-90 include bone necrosis, bone tumors, leukemia, and other hematologic dyscrasias (abnormalities).
12.190 Most of the strontium-90 in the delayed fallout is ultimately brought to earth by rain or snow, and it makes its way into the human body primarily (directly and indirectly) through plants. At first thought, it might appear that the ratio of strontium to calcium in man would be equal to that in the soil from which he obtains his food. Fortunately, however, a number of processes in the chain of biological transfer of these elements to the human body operate collectively to decrease the relative quantity of strontium that is stored in man by an overall factor of two to ten. The accumulation of strontium-90 in the human body by way of food is affected by the availability and proximity of strontium to the root system of a plant, strontium-90 uptake by the plant, transfer from plant to animal (where relevant), and transfer from plant or animal to man.
12.191 Greenhouse experiments show a slight discrimination in favor of calcium and against strontium when these elements are taken up by most plants from homogeneous soils. However, several factors make it difficult to generalize concerning the ratio of strontium to calcium in the plant compared to that in field soils. First, plants obtain most of their minerals through their root systems, but such systems vary from plant to plant, some having deep roots and others shallow roots. Most of the strontium-90 deposited in undisturbed soil has been found close to the surface, so that the uptake of this nuclide may be expected to vary with the root habit of the plant. Second, although strontium and calcium, because of their chemical similarity, may be thought of as competing for entry into the root system of plants, not all of the calcium in soil is available for assimilation. Some natural calcium compounds in soil are insoluble and are not available as plant food until they have been converted into soluble compounds. Most of the strontium-90 in the delayed fallout, however, is in a water-soluble form. Third, in addition to the strontium-90 which plants derive from the soil, growing plants retain a certain amount of strontium-90 from fallout deposited directly on the surface of the plant.
12.192 As the next link in the chain, animals consume plants as food, thereby introducing strontium-90 into their bodies. Once again, the evidence indicates that natural discrimination factors result in a strontium-90/calcium ratio in the edible animal products that is less than in the animal's feed. Very little strontium is retained in the soft tissue, so that the amount of strontium-90 in the edible parts of the animal is negligible. It is of particular interest, too, that the strontium-90/calcium ratio in cow's milk is much lower than that in the cow's feed, and thus is an important barrier to the consumption of strontium-90 by man. This barrier does not operate, of course, when plant food is consumed directly by human beings. However, it appears that about three fourths of the calcium, and hence a large fraction of the strontium-90, in the average diet in the United States is obtained from milk and milk products. The situation may be different in areas where a greater or lesser dependence is placed upon milk and milk products in the diet.
12.193 Not all of the strontium-90 that enters the body in food is deposited in the human skeleton. An appreciable fraction of the strontium-90 is eliminated, just as is most of the daily intake of calcium. But there is always some fresh deposition of calcium taking place in the skeletal structure of healthy individuals, so that strontium-90 is incorporated at the same time. The rate of deposition of both calcium and strontium-90 is, of course, greater in growing children than in adults. In addition to the fact that human metabolism discriminates against strontium, it will be noted that, in each link of the food chain, the amount of strontium-90 retained is somewhat less than in the previous link. Thus, a series of safeguards reduces deposition of strontium in human bone.
12.194 As there has been no experience with appreciable quantities of strontium-90 in the human body, the relationship between the probability of serious biological effect and the body burden of this isotope is not known with certainty. Tentative conclusions have been based on a comparison of the effects of strontium-90 with radium on test animals, and on the known effects of radium on human beings. From these comparisons it has been estimated that a body content of 10 microcuries (l microcurie is a one-millionth part of a curie, as defined in § 9.141) of strontium-90 in a large proportion of the population would produce a noticeable increase in the occurrence of bone cancer. On this basis, it has been recommended that the maximum activity of strontium-90 in the body of any individual who is exposed in the course of his occupation be taken as 2 micro curies. Since the average amount of calcium in the skeleton of an adult human is about 1 kilogram (or a little over 2 pounds), this corresponds to a concentration in the skeleton of 2 microcuries of strontium-90 per kilogram of calcium. Moreover, the limit generally considered to be acceptable for any individual member of the general population is 0.2 microcurie of strontium-90 per kilogram of calcium. The International Commission on Radiological Protection has suggested that the concentration of strontium-90 averaged over the whole population should not exceed 0.067 microcurie per kilogram of calcium.
12.195 As a result of nuclear test explosions in the atmosphere by various countries, there has been an increase in the strontium-90 content of the soil, plants, and the bones of animals and man. This increase is worldwide and is not restricted to areas in the vicinity of the test sites, although it is naturally somewhat higher in these regions because of the more localized (early) fallout.15 The fine particles of the delayed fallout descend from the stratosphere into the troposphere over a period of years, and are then brought down by rain and snow. Consequently, the amount of strontium-90 in the stratosphere available to fall on earth is determined by the difference between the quantity introduced by nuclear explosions and that removed by precipitation (and radioactive decay). This net amount reached a maximum at the end of 1962, after the cessation of nuclear weapons testing in the atmosphere by the United States and the U.S.S.R. (see Fig. 9. 143a). Subsequent additions of strontium-90 from nuclear tests made by France and mainland China have caused temporary increases in the stratospheric reservoir.
12.196 Calculations, based on somewhat uncertain premises, suggest that, in the event nuclear weapons were to be used in warfare, debris from many thousands of megatons of fission would have to be added to the stratosphere before the delayed fallout from these weapons would lead to an average concentration in the human body equal to the recommended maximum value for occupationally exposed persons, i.e., 2 microcuries of strontium-90 per kilogram of calcium.
CARBON-14 AND TRITIUM
12.197 Long-term radiation exposure can arise from carbon-14 and from tritium, the radioactive isotope of hydrogen; both of these substances are normally present in nature and they are also produced in considerable amounts in nuclear explosions. Carbon-14 is not strictly a component of fallout, but it is convenient to consider it here since it is formed by the action of fast neutrons, e.g., from a thermonuclear weapon, on nitrogen in the atmosphere (§ 9.34). Carbon-14, with a half-life of 5,730 years, emits beta particles, with the low average energy of about 0.05 MeV, an no gamma rays. Tritium is a minor product of fission, but much larger amounts are released in thermonuclear explosions (§ 9.44). The half-life of tritium is 12.3 years and the beta particles it emits have even a lower energy (average approximately 0.006 MeV) than those from carbon-14; there are also no gamma rays.
12.198 As a consequence of the testing of thermonuclear weapons, starting in 1952, there has been a large increase in the quantity of carbon-14 in the atmosphere, particularly in the stratosphere. Although this has been decreasing since 1963, there is still a significant burden of carbon-14 in the stratosphere which will find its way into the lower part of the atmosphere (troposphere). Because of its long half-life, carbon-14 decays very slowly and the decrease in concentration in the troposphere is largely due to removal of carbon dioxide by gradual solution in ocean waters.
12.199 Carbon-14 does not tend to concentrate in any particular part of the body and is distributed almost uniformly throughout soft tissue; hence, the whole body is exposed to the low-energy beta particles. The whole-body dose from carbon-14 in nature before 1952 was somewhat less than 1 millirem per annum. By 1964, this dose had been roughly doubled by the additional carbon-14 arising from nuclear tests in the atmosphere. If there are no further substantial additions, the dose will decrease gradually and approach normal in another 100 years or so. Compared with the annual radiation dose from strontium-90, mainly to the skeleton, the contribution from carbon-14 produced by thermonuclear weapons is small.
12.200 Tritium, in the form of tritiated water (§ 9.44), can enter the body by the ingestion of food and water, by inhalation of air containing tritiated water vapor, and by absorption through the skin. Since it is an isotope of hydrogen, and has the same chemical properties, tritium soon becomes distributed throughout the body wherever hydrogen is normally found. There is no reason for believing that there is any significant preferential concentration of tritium in any organ. In spite of the large increase in the quantity of tritium on the earth as a result of nuclear explosions, the annual whole-body dose was less than 0.1 millirem even at its maximum. Because of the low energy of the beta particles it emits and its relatively short half-life, tritium is much less of a long range radiation hazard than the radioisotopes already considered.
GENETIC EFFECTS OF NUCLEAR RADIATIONS
SPONTANEOUS AND INDUCED MUTATIONS
12.201 The mechanism of heredity, which is basically similar in all sexually reproducing plants and animals, including man, is somewhat as follows. The nuclei of dividing cells contain a definite number of thread-like entities called “chromosomes” which are visible under the microscope. These chromosomes are believed to be differentiated along their length into several thousands (in man) of distinctive units, referred to as “genes.” The chromosomes (and genes) exist in every cell of the body, but from the point of view of genetics (pr heredity), it is only those in the germ cells, produced in the reproductive organs (sex glands), that are important.
12.202 Human body cells normally contain 46 chromosomes, made up of two similar (but not identical) sets of 23 chromosomes each. In sexual reproduction, the first step is the union of an egg cell, produced in the ovaries of the mother, with a sperm cell, originating in the testes of the father. Each of these cells carry a set of 23 chromosomes, one representing the characteristics of the mother and the other set those of the father. The resulting fused cell then has the normal complement of 46 chromosomes. Subsequently, as the embryo develops, the cells reproduce themselves and, in general, the 46 chromosomes (and their constituent genes) are duplicated without change.
12.203 In rare instances, however, a deviation from normal behavior occurs and instead of a chromosome duplicating itself in every respect, there is a change in one or more of the genes. This change, called a “mutation,” is essentially permanent, for the mutant gene is reproduced in its altered form. If this mutation occurs in a body cell, there may be some effect on the individual, but the change is not passed on. But, if the mutation occurs in a germ cell of either parent, a new characteristic may appear in a later generation, although there may be no observable effect on the individual in whom the gene mutation occurs. The mutations which arise naturally, without any definitely assignable cause or human intervention, are called “spontaneous mutations.”
12.204 The matter of immediate interest is that the frequency with which heritable mutations occur can be in creased in various ways, one being by exposure of the sex glands (or “gonads”), i.e., testes or ovaries, to ionizing radiation. This effect of radiation has been observed with various insects and mammals, and it undoubtedly occurs also in human beings. The gene mutations induced by radiation (or by various chemicals or heat) do not differ qualitatively from those occurring spontaneously. In practice, it is impossible to determine in any particular instance if the change has occurred naturally or if it was a result of exposure to radiation. It is only the frequency with which the mutations occur that is increased by ionizing radiation. One of the concerns about radiation exposure of a large population is that there may be a substantial increase in the overall burden of harmful mutations. There would then be a greater than normal incidence of defects in subsequent generations.
12.205 All genes have the property of being either “dominant” or “recessive.” If a gene is dominant, then the appropriate characteristic affected by that gene will appear in the offspring even if it is produced by the gonads of only one of the parents. On the other hand, a particular recessive gene must occur in the gonads of both parents if the characteristic is to be apparent in the next generation. A recessive gene may consequently be latent for a number of generations, until the occasion arises for the union of sperm and egg cells both of which contain this particular gene.
BIOLOGICAL EFFECTS
12.206 As a general rule, new mutations, whether spontaneous or induced by radiation, are recessive. Nevertheless, it appears that a mutant gene is seldom completely recessive, and some effect is observable in the next generation even if the particular gene is inherited from only one parent. Furthermore, in the great majority of cases, mutations have deleterious effects of some kind. A very few of the mutations are undoubtedly beneficial, but their consequences become apparent only in the slow process of biological evolution.
12.207 The harmful effects of a deleterious mutation may be moderate, such as increased susceptibility to disease or a decrease in life expectancy by a few months, or they may be more serious, such as death in the embryonic stage. Thus, individuals bearing harmful genes are handicapped relative to the rest of the population, particularly in the respects that they tend to have fewer children or to die earlier. It is apparent, therefore, that such genes will eventually be eliminated from the population. A gene that does great harm will be eliminated rapidly, since few (if any) individuals carrying such genes will survive to the age of reproduction. On the other hand, a slightly deleterious mutant gene may persist much longer, and thereby do harm, although of a less severe character, to a larger number of individuals.
GENE MUTATIONS INDUCED BY RADIATION
12.208 Since genetic effects of radiation are not apparent in exposed individuals, information concerning mutations can be obtained only from observations on subsequent generations. Data on radiation-induced mutations are available only from laboratory studies on experimental organisms with short generation times. Unfortunately, these data cannot be extrapolated to man with any degree of certainty. The extensive investigations of genetic effects of radiation on mice appear to provide the most relevant information from which the possible effects on man may be estimated. Radiation can cause two general types of genetic change: gene (or point) mutations in which the general structure of the chromosomes remains unchanged, and chromosome abnormalities associated with gross structural changes. The former appear to be the more important and the subsequent discussion refers mainly to gene mutations.
12.209 From the earlier studies of radiation-induced mutations, made with fruit flies, it appeared that the number (or frequency) of mutations in a given population, i.e., the probability of the occurrence of mutations, is proportional to the total dose received by the gonads of the parents from the beginning of their development up to the time of conception. The mutation frequency appeared to be independent of the rate at which the radiation dose was received. The implication was that the damage to the gonads of the parents caused by radiation was cumulative with no possibility of repair or recovery. More recent experiments with mice, however, have shown that these conclusions must be revised, at least for mammals. When exposed to X rays or gamma rays, the mutation frequency in these animals has been found to be dependent on the exposure (or dose) rate at which the radiation is received. There are definite indications that some recovery can occur at low exposure rates and not too large total exposure (or doses).
12.210 For exposure rates greater than about 90 roentgens per minute, the incidence of radiation-induced mutations in male mice appears to be proportional to the total (accumulated) gamma-ray (or X-ray) exposure to the gonads; that is to say, the mutation frequency per roentgen is independent of the exposure rate.16 For exposure rates from 90 down to 0.8 roentgens per minute, however, the mutation frequency per roentgen decreases as the exposure rate is decreased. Finally, below 0.8 roentgen per minute, the mutation frequency per roentgen once again becomes independent of the exposure rate, but the value is only about one-third as large as at the high exposure rates (above 90 roentgens per minute). In other words, a given radiation exposure will produce roughly one-third as many mutations at low than at high exposure rates.
12.211 The exposure-rate effect in female mice, for radiation exposure rates of less than 90 roentgens per minute, is even more marked than in males. The radiation-induced mutation frequency per roentgen decreases continuously with the exposure rate from 90 roentgens per minute downward. At an exposure rate of 0.009 roentgen per minute, the total mutation frequency in female mice is indistinguishable from the spontaneous frequency. There thus seems to be an exposure-rate threshold below which radiation-induced mutations are absent or negligible, no matter how large the total (accumulated) exposure to the female gonads, at least up to 400 roentgens. Another important observation is that at the same high exposure rate of 90 roentgens per minute, the mutation frequency per roentgen at a total exposure of 50 roentgens is only one-third of that for a total exposure of 400 roentgens. The radiation-induced mutation frequency in female mice thus decreases both with decreasing exposure rate and with the total exposure, in the ranges studied.
12.212 For exposure to fission neutrons, no dose-rate effect has been observed for genetic mutations in male mice and only a small one in females. For large dose rates, equivalent to acute radiation exposures, the mutation frequency per rad of fast neutrons is five or six times as great as for gamma rays. It would thus appear that a RBE value of 5 or 6 should be applicable for genetic effects due to exposure to fast neutrons; but this is not strictly correct because the types of mutations induced in mice by neutron irradiation differ from those caused by X rays and gamma rays. Since there is virtually no dose-rate effect with neutrons, but a large one for X rays, the apparent RBE for neutrons becomes quite large at very low dose rates. This situation is, however, of limited interest in connection with weapons effects because neutron exposure can result only from the initial radiations and the dose rates are then in the high range.
12.213 A significant observation made with adult female mice is that a delay of at least seven weeks between exposure to a substantial dose of radiation, either neutrons or gamma rays, and conception causes the mutation frequency in the offspring to drop almost to zero. In males, on the other hand, a lengthening of the interval between exposure and fertilization of the female has little effect on the mutation frequency. It is to be noted that in this as well as other respects male and female mice exhibit different responses to radiation in the occurrence of genetic mutations. The reason is that in mice (and other mammals) the mechanisms for the development of male and female germ cells are quite different.
12.214 Since the reproductive systems are basically the same in humans as in lower mammals, it is probable that the genetic effects of radiation in man will be at least qualitatively similar to those in mice, as described above. Thus, a decrease in mutation frequency per rad is expected at very low dose rates of gamma rays in humans, especially in females, and the apparent RBE for fast neutrons should be about 5 or 6.
GENETIC EFFECTS OF NUCLEAR EXPLOSIONS
12.215 In a nuclear explosion, people would be subjected to various amounts of initial ionizing radiation, consisting of gamma rays and neutrons, delivered at a high dose rate, and also possibly to the beta particles and gamma rays from fallout received at a very much lower dose rate. Because of interbreeding between exposed and unexposed persons, it is not possible to make accurate predictions of the genetic consequences. A rough estimate is that an acute dose of about 50 rems to the gonads of all members of the population would result in additional mutations equal to the number occurring spontaneously. But this may not allow for the possible advantage that might arise from delaying conception for some months after exposure to radiation. Although, to judge from the observations on mice, this might not decrease the genetic effects of radiation in males, recovery in the female members of the population would bring about a substantial reduction in the “load” of mutations in subsequent generations.
12.216 Gamma rays from radionuclides of short half-life in the early fall out on the ground or in the surroundings will be part of the initial (acute) radiation dose to the gonads. Beta particles and gamma rays emitted from constituents of the fallout that enter the body and remain there for some time can also induce mutations. Genetic effects of strontium-90 are expected to be relatively minor. The element strontium tends to concentrate in the skeleton and because of the short range of the beta particles from strontium-90 in the body, they do not penetrate to the gonads. Furthermore, the intensity of the secondary X radiation (bremsstrahlung) produced by the beta particles is low. Finally, the amount of strontium-90 in soft tissue, from which the beta particles might reach the reproductive organs, is small. Radioiodine in the body would also not be important because all isotopes of iodine are taken up quite rapidly by the thyroid gland and the exposure of the gonads would be insignificant.
12.217 Cesium-137,carbon-14, and tritium are in a different category as internal sources because they are distributed throughout the body and so can cause irradiation of the gonads. Moreover, the decay of cesium-137 is accompanied by gamma rays of fairly long range.17 From the standpoint of the genetic impact of a particular radionuclide, the total radiation dose to the population over many generations must be taken into account. Hence, although the annual radiation dose to the gonads from carbon-14 is less than from cesium-137, the overall effect of these two substances may not be very different because of the much longer half-life of carbon-14. An additional effect can result from the radioactive decay of carbon-14 atoms in the molecules that carry genetic information. Replacement of a carbon atom by its decay product, nitrogen-14, would result in a change in the nature of the molecule.
12.218 The suggestion has been made that tritium may become concentrated in the genetic molecules and so represent a special hazard. There is, however, no convincing evidence that such is the case. It appears that the increase in mutation frequency that might arise from the presence of tritium in the gonads is not appreciably greater than would be expected from the dose to the body as a whole. Since the whole body dose from tritium produced in a nuclear explosion is less than from carbon-14 and the effective half-life is considerably shorter, the genetic effects of tritium should be very much less than those of carbon-14.
12.219 In attempting to assess the genetic effects of internal radiation emitters, it should be borne in mind that, although the total radiation doses over many generations may be large, the dose rate is very low. In fact, it may be so low that the effects in females, in particular, will be negligible. Furthermore, the radiation from fallout should be compared with the gonad exposure of all members of the population to the natural background radiation, i.e., apart from that due to nuclear explosions. In the United States, the average dose to the gonads, from cosmic rays and from radioactive isotopes in the body (especially potassium-40) and in the ground, is about 90 millirems per annum. It has been estimated that the fallout from explosions of a few hundred megatons yield would be necessary to double the overall mutation rate arising from background radiation. This, incidentally, represents probably only a small fraction of the total number of spontaneous mutations.
PATHOLOGY OF ACUTE RADIATION INJURY18
CELLULAR SENSITIVITY
12.220 The discussion presented in § 12.90 et seq. has been concerned chiefly with general symptoms and the clinical effects of radiation injury. These effects are due directly to the action of nuclear radiation upon individual organs and tissues. The changes in the peripheral blood, for example, reflect the damage done by nuclear radiation to the bone marrow and lymphatic tissue. The pathologic changes in other systems and organs caused by ionizing radiation, which are the basis of the clinical radiation syndrome, are discussed here briefly.
12.221 Radiation damage is the result of changes induced in individual cells. Morphologically demonstrable changes, such as chromosome breaks, nuclear swelling, increased cytoplasmic viscosity, cellular permeability, and cellular death, are manifested as altered bodily functions when enough cells are affected to reduce the total function of the organ made up of these cells. In certain instances, cells may be killed outright with very high doses of radiation (interphase death), but more commonly irradiated cells die when they divide to reproduce (mitosis-linked death). Delayed death of this kind may occur after several cell divisions following irradiation, so that the effect may not be observed until some time after exposure. Mitosis-linked death is apparently caused by chromosomal and perhaps other nuclear abnormalities, but with time some of these abnormalities are repaired. Consequently, the longer the time between cell divisions, the greater is the opportunity to recover from radiation damage. Cells of different types and organs have quite different degrees of radiosensitivity based mainly on the rapidity of the cell division. Chromosomal changes can also occur that will not result in cell death but in heritable abnormalities (§ 12.208) or in cell transformations which may lead to cancer.
12.222 Of the more common tissues, the radiosensitivity decreases roughly in the following order: lymphoid tissue, bone marrow, gastrointestinal epithelium, germinal epithelium of the gonads, embryonic tissues, corneal tissue, endothelial cells of the blood vessels, germinal epithelium of the skin, differentiated nervous tissue, collagen and elastic tissue, and bone and cartilage. The lymphocytes are remarkable in that they are killed by relatively small acute radiation doses (see below).
LYMPHOID TISSUE
12.223 Lymphoid tissue is composed of the lymph nodes, tonsils, adenoids, spleen, and the submucosal islands of the intestine. The lymphocytes of the peripheral blood arise in these various sites. Wherever these cells occur, they are the most radiosensitive cells of the whole body. In fact, lymphocytes are killed outright by radiation doses as low as 100 rems or less.
12.224 Under the microscope, irradiated lymphocytes can be seen to be undergoing pyknosis and subsequent disintegration. As these cells die, their remnants are removed and the lymph nodes atrophy. This change was common among the victims of the nuclear bombs in Japan. After damage by radiation, the lymph nodes do not produce new lymphocytes for periods that vary with the radiation dose. As a result of this cessation of production, combined with death of circulating lymphocytes, there is a rapid fall in the number of the latter. This easily measurable early change in the peripheral blood has been found to be a useful means of prognosis following radiation exposure. A rapid, almost complete, disappearance of lymphocytes implies that death is highly probable, whereas no change within 72 hours is indicative of an inconsequential exposure.
12.225 Atrophic lymph nodes, tonsils, adenoids, Peyer's patches of the intestine, appendices, and spleens were common findings among the radiation casualties in Japan.
BONE MARROW
12.226 Since all the other formed blood cells, except the lymphocytes, arise from radiosensitive marrow cells, the acute radiation exposure syndrome is accompanied by severe changes in cellular composition of the blood. Under normal circumstances, the mature blood cells leave the marrow and enter the bloodstream where they remain until destroyed by natural processes and in defense against infection. The different kinds of cells have different spans of natural life. The shorter the life of a particular cell, the more quickly will radiation damage to the parents of that particular cell be revealed by a decrease in the number of such cells in the circulation. The red blood cells, which have the longest life span (about 120 days), are the last to show a reduction in number even though their parent cells, the erythroblasts, are almost as radiosensitive as the lymphocytes.
12.227 Bone marrow exhibits striking changes soon after irradiation. There is at once a temporary cessation of cell division. Those cells in the process of dividing go on and complete the process, after which all the cells in the marrow mature progressively. Since they leave the marrow as rapidly as maturity is reached, the marrow becomes depleted at once of both adult and less mature cells. As time passes, the marrow, barring regeneration, becomes progressively more atrophic until in the final stage it consists of dilated blood filled sinuses, with gelatinous edema of the spaces left empty by the loss of marrow cells, and large macrophages containing the debris of dead cells removed from the circulation. Such extreme atrophy of the marrow was common among those dying of radiation injury in Japan up to 4 months after exposure. In some of these delayed radiation deaths, the bone marrow showed a return of cellular reproductive activity.
HEMORRHAGE AND INFECTION
12.228 Hemorrhage is a common phenomenon after radiation exposure because the megakaryocytes, from which the blood platelets necessary for clotting are formed, are destroyed and platelets are not replenished. If hemorrhage occurs in vital centers, death can result. Often the hemorrhages are so widespread that severe anemia and death are the consequences.
12.229 The loss of the epithelial coverings of tissues, together with the loss of white blood cells and the decreased ability to produce antibodies, lowers the resistance of the body to bacterial and viral invasion. If death does not take place in the first few days after a large dose of radiation, bacterial invasion of the blood stream usually occurs and the patient dies of infection. Often such infections are caused by bacteria which, under normal circumstances, are harmless.
12.230 Very often in whole-body irradiation the outward signs of severe damage to the bone marrow, lymphatic organs, and epithelial linings are gangrenous ulcerations of the tonsils and pharynx. This condition (agranulocytic anemia) is also found in cases of chemical. poisoning of the bone marrow that resembles the effect of radiation exposure. Such ulcerations and the pneumonia that often accompanies them are unusual in the respect that very little suppuration is found because of the paucity of leucocyte cells. Although most of the bacteria in such ulcerations can usually be controlled by antibiotic drugs, the viruses and fungi which also invade such damaged tissues are not affected by treatment, and fatal septicemia is common.
REPRODUCTIVE ORGANS
12.231 Cell division in the germinal epithelium of the testes stops at once with lethal exposure to ionizing radiation. The first change is pyknosis or nuclear death of the spermatogonia, the most primitive of the male germinal epithelium. Following this change, the more developed cells undergo maturation without further division, so that the testicular germinal cells leave the testes as adult sperm, and the most primitive cells disappear sequentially as they mature and die during cell division.
12.232 Changes in the ovaries caused by radiation are less striking than those in the testes. The primordial ova can be found in progressive stages of post irradiation atrophy and degeneration. In some Japanese irradiation victims, the ovarian follicles failed to develop normally and menstrual irregularities resulted. There was an increased incidence of miscarriages and premature births, along with an in creased death rate among expectant mothers. These changes were related to the radiation dose, as determined by the distance from ground zero.
12.233 Morphologic changes in the human reproductive organs, compatible with sterility, are thought to occur with doses of 450 to 600 rems. Various degrees of temporary sterility were found among surviving Japanese men and women. Many supposedly sterile from exposure to significant doses of radiation have since produced children who are normal by ordinary measurements.
LOSS OF HAIR
12.234 Epilation was common among exposed Japanese surviving more than 2 weeks after the explosion. The onset of epilation from the head was between the 13th and 14th days after exposure in both sexes. Combining accentuated this change, although copious amounts of hair were lost spontaneously for about 2 weeks. The distribution of the radioepilation conformed in general with that expected from the senile changes of male ancestors. The hair of the eyebrows, eyelashes, and beard came out much less easily than from the head. In severely exposed but surviving cases, hair began to return within a few months, and epilation was never permanent.
GASTROINTESTINAL TRACT
12.235 Some of the first gross changes noted in radiation-exposed Japanese were ulcerations of the intestinal lining. The mucosa of the first part of the small intestine is the most radiosensitive but usually does not ulcerate deeply. Ulcers are most commonly found after irradiation in the lymphoid tissues of the lower ileum and in the caecum, where bacterial invasion is common.
12.236 Microscopically profound changes are found throughout the gastrointestinal tract. For example, the acid-secreting cells of the stomach are lost. Mitosis stops in the crypts of the intestinal glands and, as a result, that cells covering the villi of the intestine are not replaced and the villi become swollen, turgid, and denuded. When bacterial invasion occurs, ulcers covered by a shaggy, fecally contaminated, exudate develop. Since the white blood cells are simultaneously depleted and too few in number to combat infection these intestinal ulcerations are often the point of entry of bacteria that kill the victim of heavy radiation exposure.
NERVOUS SYSTEM
12.237 Although certain nerve cells are among the most radioresistant cells in the adult body, the nervous tissue of the embryo and some cells of the adult cerebellum are relatively sensitive to radiation. Early disorientation and con may be induced by brain damage at dose levels of thousands of rems.
BLAST-RELATED EFFECTS
VELOCITIES OF GLASS FRAGMENTS
12.238 Glass fragments produced by air blast are a substantial hazard and the injuries they can cause are related to the velocities attained (§ 12.42). Measurements have been made of the fragments produced from glass panes, mounted in either steel or wood frames, when destroyed by the blast from nuclear (11 to 29 kilotons) or conventional (15 to 500 tons) explosions. The types of glass ranged from 0.25-inch thick plate glass, through various standard thicknesses of single- and double strength glass, to thin nonstandard panes 0.064 inch thick. The results obtained can be represented, with an accuracy of roughly ± 10 to 15 percent, by the straight line in Fig. 12.238. The geometric mean velocity, i.e., the antilogarithm of the mean of the logarithms of the velocities, represented by V50 feet per second, is modified by an empirical scaling factor for the thickness (t inches) of the glass panes. The effective peak overpressure (pounds per square inch) is equal to the peak reflected overpressure if the glass is oriented face-on to the blast wave and it is the same as the incident peak overpressure if the pane is located on the side or back of a structure relative to the advancing shock front. From Fig. 12.238 the geometric mean velocity of the fragments can be determined for glass panes of any specified thickness exposed to a given effective peak overpressure.
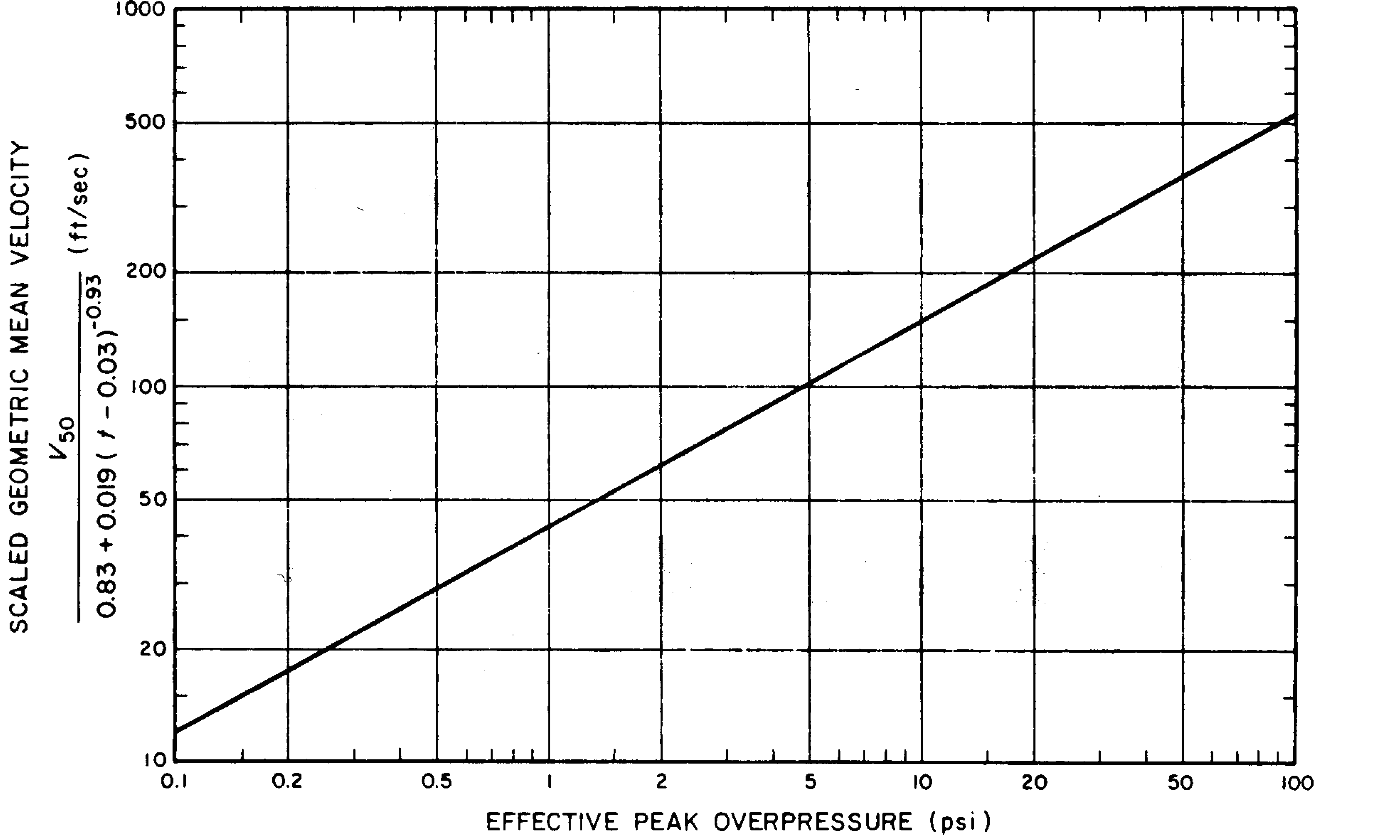
DECELERATIVE TUMBLING
12.239 The results of the tests referred to in § 12.45, on the decelerative tumbling of various animal cadavers dropped onto a hard, flat surface at different velocities, are represented graphically in Fig. 12.239; the possible error is within the range of about ± 10 to 15 percent. The initial velocity V; feet per second and the stopping distance S feet are scaled for the mass of the animal (m pounds). Scaled stopping times are also shown. Thus, for a given initial velocity and animal mass, the stopping distance for decelerative tumbling may be derived directly from the linear plot and the corresponding stopping time may be determined by interpolation. Although the data were obtained from tests with animals, it is thought that the results can be applied to the decelerative tumbling of humans provided there is no significant bouncing.
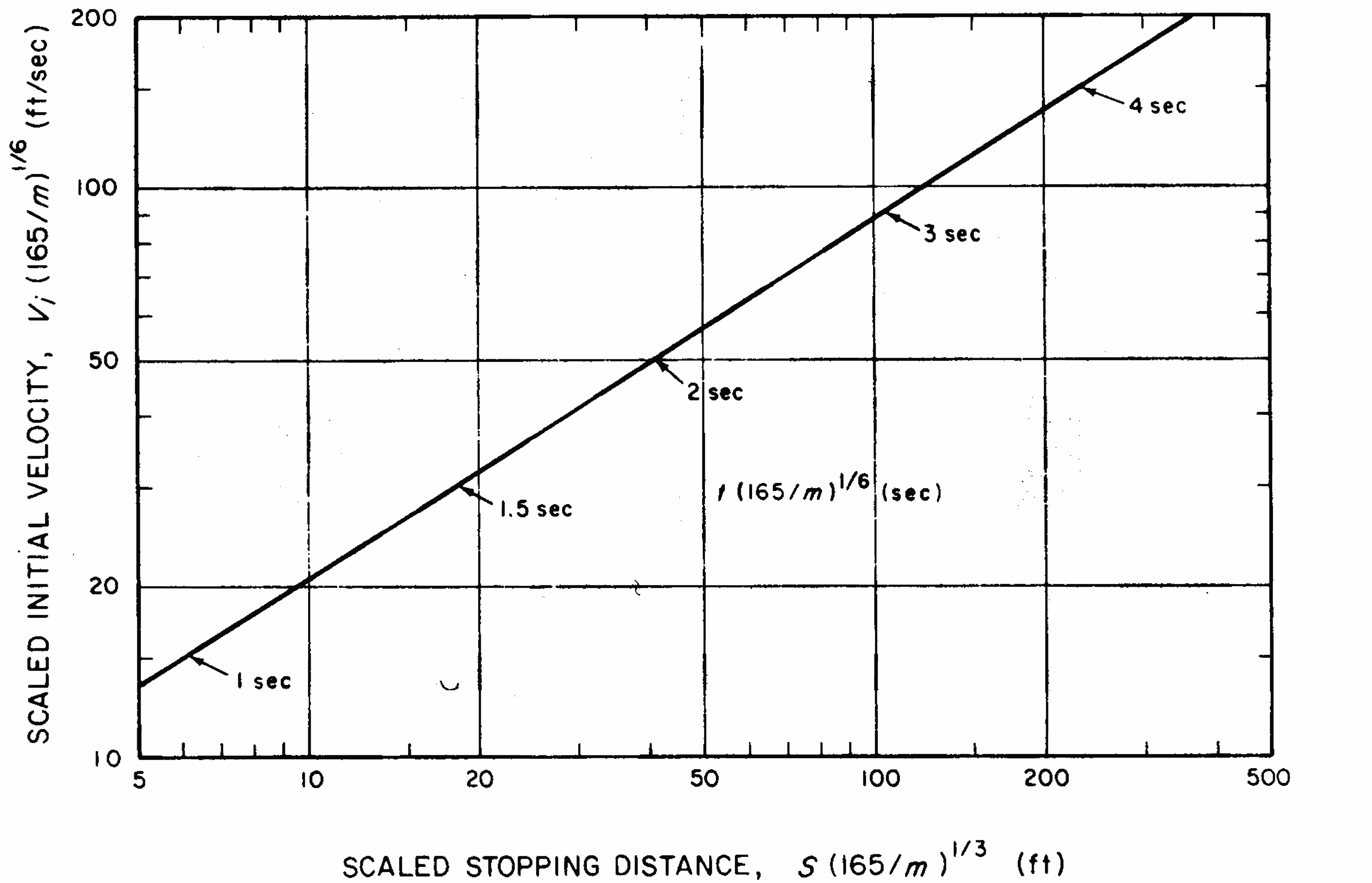
EFFECTS ON FARM ANIMALS AND PLANTS
INTRODUCTION
12.240 In general, the three main immediate physical effects of nuclear explosions, i.e., blast, thermal radiation, and the initial nuclear radiation, have similar potential for causing damage to animals and plants as they do to humans. As biological systems, larger animals are similar to man and would experience much the same blast, burn, and radiation injuries, if exposed in the same manner. In fact, much information concerning the expected effects of nuclear weapons on man, apart from the data from Japan, has been inferred from studies on animals. Plants, on the other hand, vary greatly in the characteristics that determine injury from the immediate physical effects of nuclear explosions. Consequently, for plants the range of biological responses is greater than for man or animals.
12.241 In nuclear warfare, an important need would be to assure an adequate food supply for the survivors, especially during the early post-attack period. The main concern would then not be with the immediate effects of the explosions, but rather with the effects of the fallout on farm animals and crop plants forming part of man's food chain. As a rule, the seriousness of these effects increases with increasing dose and dose rate of ionizing radiations. The total effect of a given dose is also influenced by the stage of development of the organism and the environmental conditions prior to, during, and after the exposure. As with the immediate effects of a nuclear explosion, plants show a wide range of sensitivity to fallout radiation.
12.242 Another factor to be considered is the possible consequences of the accumulation of various radioisotopes in food supplies due to the residual radioactivity in soils and water from deposited worldwide fallout. However, these effects are of a protracted nature and their importance is not well understood at present.
12.243 Another matter of interest in connection with the effects of nuclear explosions on plants and farm animals is the possibility of serious ecological disturbances. These might be caused by large-scale fires, denuding of forests by fallout, destructive plagues of insects which are known to be relatively insensitive to radiation, and so on. It is not expected that such effects would be severe enough to prohibit or seriously delay recovery of food production facilities after a nuclear attack.
FALLOUT RADIATION EFFECTS ON LIVESTOCK
12.244 As with man, fallout may cause both internal and external radiation exposures to animals. The external (whole-body) exposure would arise mainly from gamma rays, and if the fallout particles should remain on the skin for some time, the animals could suffer beta burns (§ 12.155). Internal radiation exposure could result from farm animals consuming contaminated grass and thereby ingesting fallout particles. Beta radiations from these particles would then irradiate the walls of the intestinal tract whereas the gamma rays would contribute to the whole-body exposure. Certain radioisotopes may be leached from the fallout particles and enter the bloodstream; they may then be deposited in specific parts of the body, e.g., iodine in the thyroid and strontium in the skeleton.
12.245 Skin injury caused by fall out was observed in cattle exposed at the TRINITY test (§ 2.36) and also in animals during atmospheric tests at the Nevada Test Site. Minor to severe injuries due to beta radiation have occurred, although none of the cattle died within 150 days of exposure. The skin injuries appeared to be similar to thermal burns except that the latter are soon visible whereas the effects of beta particles may not be seen for three or four weeks.
12.246 The damage to the cattle at the TRINITY site was described as the development of zones of thickened and hardened skin which appeared as plaques and cutaneous horns. After 15 years, three of the exposed cows developed scale-like carcinomas of the skin in the affected regions, but it is not entirely clear that they were induced by radiation. In areas less severely affected, there was some loss and graying of the hair. The location of these cattle with respect to ground zero is not known, but it is estimated that the whole-body gamma radiation dose was about 150 rems, although the skin dose may have been very much larger. There was no evidence of radiation damage on the lower surfaces of the body that might have been caused by exposure from fallout on the ground.
12.247 Information concerning the possible effects of fallout on farm animals under various conditions has been obtained from studies with simulated fallout sources. Three main situations of interest, depending on the location of the animals, are as follows:
- In a barn: whole-body exposure to gamma rays from fallout on the roof and the surrounding ground.
- In a pen or corral: whole-body exposure to gamma rays from fallout on the ground and exposure of the skin to beta particles from fallout deposited on the skin.
- In a pasture: whole-body exposure to gamma rays from fallout on the ground, exposure of the skin to beta particles, and exposure of the gastrointestinal tract from fallout on the grass.
The exposure to gamma rays is simulated by means of an external cobalt-60 source. Skin irradiation is achieved by attaching to the back of the animal a flexible source of beta particles. Finally, the internal exposure is simulated by adding to the animal's feed a material consisting of yttrium-90 fused to 88-175 micrometers particles of sand, giving a specific activity of 10 microcuries per gram of sand. This product is considered to be representative of the beta radiation from the fallout produced by a land-surface detonation.
12.248 Observations have been made on animals exposed to whole body gamma radiation alone (barn) or in combination with skin exposure (pen or corral) or with exposure of the skin and the intestinal tract (pasture) at dose rates of the order of magnitude expected from fallout. From the results, estimates were made of the total gamma exposure which would be fatal to 50 percent of a large group of animals within 60 days (LD50/60); the values are summarized in Table 12.248. It is evident that, for cattle and sheep, which are ruminants, internal exposure can contribute substantially to the lethality of fallout.
Total Gamma Exposure (roentgens) | |||
---|---|---|---|
Animals | Barn | Pen or Corral | Pasture |
Cattle | 500 | 450 | 180 |
Sheep | 400 | 350 | 240 |
Swine | 640 | 600* | 550* |
Horses | 670 | 600* | 350* |
Poultry | 900 | 850* | 800* |
* No experimental data available; estimates are based on grazing habits. anatomy. and physiology of the species
12.249 The data in the table apply to extreme conditions and are intended only to indicate the different sensitivities to radiation of a few animal species, the kinds of doses that might prove fatal, and the effects of combining different types of exposures. The basic assumption involved is that the animals remain in a given situation while they accumulate an exposure of a few hundred roentgens of radiation.19 In practice, of course, the animals would probably be removed as soon as possible from a contaminated area and, in any event, contaminated grass would soon be replaced by clean fodder. Swine are normally fed in a drylot and would probably not ingest enough radioactivity to increase doses above those expected from whole-body irradiation alone.
12.250 There are considerable variations in radiation sensitivity in a given animal species, just as in man, but on the whole it appears, in agreement with the estimates in Table 12.248, that cattle and sheep are more sensitive to radiation than are swine. Furthermore, among those who survive, the recovery time is shorter in swine than in cattle and sheep. As a general rule, young animals are more sensitive to radiation than are adults, but the difference appears to be less marked for swine than for cattle. At high exposure rates, the total exposure required to produce a certain degree of lethality is smaller than when the rate is low, suggesting the possibility of partial recovery by the animal from radiation injury. Again, this effect is more marked for swine than for other livestock.
12.251 The great majority of farm animals receiving an exposure of less than 400 roentgens of whole-body radiation alone would be expected to survive. However, they will show symptoms similar to those observed in man. The primary symptoms are those associated with damage to the blood-forming tissues; they usually include a severe drop in the number of platelets in the blood and gastrointestinal damage caused by failure in blood clotting. Increased permeability of the capillaries also contributes to the loss of blood cells, plasma, and electrolytes (salts). Most of these losses occur between 14 and 30 days after exposure, at which time the white-cell count is low; fever and bacterial invasion may also occur.
12.252 Cattle receiving whole-body exposures in the range of 200 to 600 roentgens commonly experience some loss of appetite and slight fever for about 24 hours. They then appear normal for about 14 days (latent stage), after which there is a marked fever in those receiving the larger radiation exposures; most of the latter will die within a month or so. Those animals which survive show only a mild fever. Very few, if any, of these survivors are expected to suffer the serious loss of appetite and vomiting which are associated with the gastrointestinal radiation syndrome at higher exposures.
12.253 The effects of internal radiation exposures have been studied by adding the simulant mentioned in § 12.247 to the feed of sheep. The earliest symptoms were loss of appetite, diarrhea, weight loss, and fever. The sandy radioactive material tended to collect in “pockets” in the rumen and abomasum20 where the radiation caused ulceration and accumulation of fibrinous exudate. No gross lesions were found in the intestines of sheep under these conditions. Loss of appetite was accompanied by stagnation in the rumen which prevented the normal passage of the animal's food. This was followed by severe diarrhea and weight loss. Sheep that survived usually returned to normal feed consumption within 60 days, but considerably more time was required to recover the loss of weight.
12.254 Whole-body exposure to 240 roentgens of external gamma radiation, at the high exposure rate of 60 roentgens per hour, affected neither the body weight nor the feed consumption of sheep and cattle. If the whole-body exposure was supplemented by a skin dose there was some decrease in weight, and an even greater decrease if there was also exposure of the intestinal tract. However, it appears that at radiation doses below lethal values and at dose rates expected from fallout, the effect on livestock production would be minor at most. As a rule, irradiated dairy cows produce as much milk as those which have not been exposed, but lactation may be reduced as a result of destruction of thyroid tissue by radioiodine from ingested fallout. It is possible that the concentrations of strontium-90 and of iodine-131 may make the milk unsuitable for general use. Most sheep, cattle, and swine surviving the exposure to fallout, even those with gastrointestinal tract injuries from ingested fallout, could eventually be used for food under emergency conditions. Until more data are available, it has been recommended that, for 15 to 60 days after exposure to radiation levels that might cause some mortality, only muscle meat from surviving animals be used for food.
FALLOUT RADIATION EFFECTS ON PLANTS
12.255 Plants differ from animals (and man) with respect to radiation exposure from fallout; animals can move or be moved from the fallout field whereas plants in the ground must remain in the same location during their lifetime. Since food crops are harvested at the end of the growing season, the total exposure received will be greatly dependent on the stage of development at which the fallout occurs. Thus, a young seedling will receive a much larger radiation dose than will a fairly mature plant which is almost ready for harvesting. Furthermore, the sensitivity of a plant to radiation is different at different growth stages. These and other factors make it impossible to present any precise information concerning the expected effects of fallout on plants. Nevertheless, some general conclusions can be drawn.
12.256 At sufficiently large doses, radiation can seriously reduce the growth and yield of a plant, particularly if it is exposed at certain stages of development. In addition, there may be loss of reproductive capacity, changes in shape and appearance, wilting, and ultimately death. There may also be changes in the normal plant tolerance to environmental stresses. The sensitivities of plants to radiation vary over a wide range and they are influenced by many biological, environmental, and radiological factors. The sensitivities of different species may differ as much as 100-fold or more, and there may be a 50-fold range of sensitivity in a given species at different stages of growth. Thus, certain stages of the development of reproductive structures, e.g., formation of flower buds, are very sensitive to radiation, but the ripe seeds are much more resistant.
12.257 As with animals, the response of a plant to a given dose of radiation depends on the dose (or exposure) rate, although the effect appears to be more marked for plants. A much larger total dose is usually required to produce a given degree of injury to plants when the dose rate is low than when it is high. At very high and very low dose rates, however, there is no observable evidence of a dose-rate effect.
12.258 Among the many important environmental conditions influencing the radiation response of plants are climate, temperature, light, soil moisture, and competition from other plants. Excluding the effects of drought, changes in environmental factors can result in as much as a ten-fold change in apparent radiosensitivity of a given plant species. Significant exposures to radiation are expected to delay flower initiation and fruit ripening. Hence, plants with a growing season that is limited by climatic conditions, e.g., tomato, may survive through the growing season but would produce essentially no useful yield.
12.259 Since seeds are needed to provide the next crop, the viability of seeds from irradiated plants is important. Adverse characteristics are sometimes present, although the seed appears to be normal. Too little is known about this matter for any definite statements to be made. Seeds already formed are fairly resistant to radiation and seeds in storage will probably remain essentially unaffected. Seed potato tubers and small onion transplants are more sensitive than ordinary dry seeds. Exposure of seeds to sufficiently large doses of radiation is known to produce mutations, and mutations may well appear in seeds from exposed plants. Although most of the mutations are deleterious, a number of beneficial mutant forms have been developed from irradiated seeds.
12.260 Information on the effects of actual fallout on plants is meager. At the Nevada Test Site, trees and shrubs have been killed by radiation from fallout, but the plants have been close to the locations of cratering explosions. Substantial amounts of fallout particles were deposited on the leaves where they remained for some time because of the small rainfall in the desert area. No such occurrences have been observed following contained, buried nuclear explosions. Essentially all that is known about the effects of radiation on plants has been obtained from tests in which plants at various stages of growth and development have been exposed to gamma rays from an external source.
12.261 Because of the great variations in radiosensitivity even among plants of the same species, the results obtained with experimental plants are applicable only under the precise conditions of the experiments. However, an important conclusion has emerged from these studies which should provide a general guide as to the expected effects of gamma radiation on plants. At equivalent growth stages and under similar conditions, the radiosensitivity of a plant is directly related to the size of the chromosomes, measured as the average volume occupied per chromosome in the cell nucleus. The larger the effective chromosome volume, the more sensitive the plant is to radiation. In other words, under equivalent conditions, a given total dose (or exposure) of radiation will cause a larger proportion of deaths and a greater decrease in yield from the surviving plants, the larger the chromosome volume. Or stated in another way, the larger the chromosome volume, the smaller the radiation dose required to produce a given degree of damage to the plants.
12.262 On the basis of chromosome volume (and experimental observations of lethality and yield) some important food crops can be placed in an approximate order of decreasing sensitivity to radiation as follows: onions, small-grain cereals, e.g., wheat, barley, oats, and corn (but not rice), field peas, lettuce, lima beans, potatoes, sugar beets, broccoli, and rice. It is of interest that young seedlings of rice appear to be exceptionally resistant to radiation. Even for the least resistant plant species, a total exposure of about 1,000 roentgens (or more) in the seedling stage is required to kill about 50 percent of the exposed plants, whereas for the most radioresistant plant 15,000 roentgens may be required. The decrease in yield of the surviving plants after exposure to radiation follows the same order, in general, as the increase in lethality.
12.263 Although woody plants are not a source of food, they are of economic importance. Evergreen trees (gymnosperms), such as pines and related species, are quite sensitive to radiation. Deciduous trees, which shed their leaves at the end of each growing season, are much less sensitive; the exposures that will kill about half the exposed trees range from 2,600 to 7,700 roentgens. However, even smaller exposures would have a serious effect on the economic value of these trees.
12.264 The results described above refer to exposures from gamma radiation. In a fallout situation, however, the plant would be subjected to beta radiation in addition. In fact, it appears that for many crop plants, which typically have relatively little tissue mass around their most radiosensitive parts and which are often in contact (or near contact) with the fallout particles, the dose from beta radiation may be greater than from gamma rays. This would be particularly the case in the early stages of plant growth. Beta radiation may thus make an important contribution to the injury of plants and may be the dominant cause of damage in many situations. Apart from the view that beta and gamma radiation have equivalent effects for the same dose in rads, information concerning beta-radiation injury and the possible synergism with gam:na radiation is very sparse.
12.265 Food crops harvested from plants that have survived exposure to fallout would probably be safe to eat under emergency conditions, especially if the exposure occurred during the later stages of growth. Care would have to be taken to remove by washing any fallout particles that might be attached to leaf or root vegetables. The major problem would arise from the possible presence in the edible parts of the plant of radionuclides taken up from the soil by the roots or from particles deposited on the leaves. Because of the complexities involved, no generalizations can be made and each situation would have to be evaluated individually.
BIBLIOGRAPHY
- et al., “The Calculation of Retinal Burn and Flashblindness Safe Separation Distances,” U.S. Air Force School of Aerospace Medicine, September 1968, SAM–TR– 68–106.
- , “Plutonium: Biomedical Research,” Science, 183, 715 (1974).
- , “Effects of Feeding Yttrium-90 Labeled Fallout Simulant to Sheep,” Radiation Research, 43, 71 (1970).
- , “Survival of Food Crops and Livestock in the Event of Nuclear War,” Proceedings of a Symposium held at Brookhaven National Lab oratory, September 15–18, 1970, AEC Symposium Series, No. 24, U.S. Atomic Energy Commission, 1971.
- , “The Influence of External Body Radiation on Mortality from Thermal Burns,” Annals of Surgery, 136, 533 (I 952).
- , “Beta Dosimetry for Fallout Hazard Evaluation,” Stanford Research Institute, Menlo Park, California, July 1970, EGU–8013.
- . “The Acute Radiation Syndrome: Y-12 Accident,” Oak Ridge Institute of Nuclear Studies, April 1959, ORINS–25.
- et al., U.K. Atomic Energy Authority Report AERE HP/R 2353 (1957); “Strontium in Diet,” Brit. Medical Journal, 1, 1371 (1958).
- et al., “A Twenty-Year Review of Medical Findings in a Marshallese Population Accidentally Exposed to Radioactive Fallout,” Brookhaven National Laboratory, September 1975, BNL 50424. (This report contains references to previous studies.)
- et al., “Leukemia in Atomic Bomb Survivors, Hiroshima and Nagasaki,” Radiation Research, 45, 216 (1971).
- et al., “Cancer in Japanese Exposed as Children to Atomic Bombs,” The Lancet, May 8, 1971, p. 927.
- , “Studies of the Mortality of A-Bomb Survivors, 5. Radiation Dose and Mortality, 1950–1970,” Radiation Research, 50, 649 (1972).
- , “Strontium-90 in Man: IV,” Science, 132, 448 (1960).
- , “Fallout Simulant Development: Leaching of Fission Products from Nevada Fallout and Properties of Iodine-tagged Simulant,” Stanford Research Institute, Menlo Park, California, June 1970, SRl–7968. (This report contains references to and summaries of previous studies.)
- , “Radiobiological Factors in Manned Space Flight,” Chapters 5 and 6, National Academy of Sciences–National Research Council, Publication No. 1487, 1967.
- , “Analysis of the First Thermal Pulse and Associated Eye Effects,” Aerospace Medical Research Laboratories, Wright Patterson Air Force Base, Ohio, December 1968, AM RL–TR–67–214.
- , “The Entry of Fission Products into Food Chains,” Progress in Nuclear Energy, Series VI, Vol. 3., Pergamon Press, Inc., 1961.
- , “Introduction to Long-Term Biological Effects of Nuclear War,” Stanford Research Institute, Menlo Park, California, April 1966, MU–5779.
- MILLER, R. W., “Delayed Radiation Effects in Atomic Bomb Survivors,” Science, 166, 569 (1969).
- , “The Biological Effects of Atomic Radiation,” 1956 and 1960; “Pathological Effects of Atomic Radiation,” Publication No. 452, 1961; “Effects of Inhaled Radioactive Particles,” Publication No. 848, 1961; “Long Term Effects of Ionizing Radiations from External Sources,” Publication No. 849, 1961; “Effects of Ionizing Radiation on the Human Hematopoietic System,” Publication No. 875, 1961; “The Effects on Populations of Exposure to Low Levels of Ionizing Radiation,” 1972 (a complete review with numerous references to the biological effects of ionizing radiations); “Long-Term Worldwide Effects of Multiple Nuclear–Weapons Detonation,” 1975; Washington, D.C.
- , “Basic Radiation Protection Criteria,” NCRP Report No. 39, Washington, D.C., 1971.
- , “Medical Effects of the Atomic Bomb in Japan,” National Nuclear Energy Series VIII, McGraw-Hill Book Co., Inc., 1956.
- , “Clinical Radiation Pathology,” Vols. I and II, W. B. Saunders Company, 1968.
- , “The Effects of Radioactive Fallout on Food and Agriculture,” North Atlantic Treaty Organization Report (1971).
- , “The Effects of External Gamma Radiation from Radioactive Fallout on Plants with Special Reference to Crop Production,” Radiation Botany, 11, 85 (1971).
- , “Report of the United Nations Scientific Committee on the Effects of Atomic Radiation,” A/5216 (1962); A/5814 (1964); A/6314 (1966); A/7613 (1969); A/8725 (1972); United Nations, New York.
- et al., “Comparative Nuclear Effects of Biomedical Interest,” Civil Effects Study, U.S. Atomic Energy Commission, January 1961, CEX–58.8.
- et al., “The Relation Between Eardrum Failure and Blast-Induced Pressure Variations,” Space Life Sciences, 2, 158 (1970).
- , “The Nature of Problems Involved in Estimating the Immediate Casualties From Nuclear Explosions,” Civil Effects Study, U.S. Atomic Energy Commission, July 1971, CEX 71.1.
- et al., “The Biodynamics of Air Blast,” Advisory Group for Aerospace Research and Development, North Atlantic Treaty Organization, December 1971, AGARD–CP– 88–71, p. 14–1. Also published as DASA 2738T, July 1971. (A complete review with numerous references.)
- et al., “Thyroid Cancer in Atomic Bomb Survivors, Hiroshima and Nagasaki,” Amer. J. Epidemiology, 89, 4 (1969).
*These documents may be purchased from the National Technical Information Service, Department of Commerce, Springfield, Virginia, 22161.
ENDNOTES
- 1 Computed from data in A. W. Oughterson and S. Warren (Editors), “Medical Effects of the Atomic Bomb in Japan,” McGraw-Hill Book Co., Inc., Chapter 4, 1956. For further information, see also “Medical Effects of the Atomic Bomb,” Report of the Joint Commission for the Investigation of the Effects of the Atomic Bomb in Japan, Office of the Air Surgeon NP–3041; “Medical Report on Atomic Bomb Effects,” The Medical Section, Special Committee for the Investigation of the Effects of the Atomic Bomb, National Research Council of Japan, 1953; and the U.S. Strategic Bombing Survey, “The Effects of Atomic Bombs on Hiroshima and Nagasaki,” 1946. [ref. § 12.09] X
- 2 The word “geometry” is used here as a general term to describe the location of an individual in relation to the details of the environment that may affect the blast wave characteristics. [ref. § 12.35] X
- 3 The acceleration coefficient is the product of the projected area presented to the blast wave and the drag coefficient (§ 4.19) divided by the mass of the object. [ref. § 12.41] X
- 4 Figures represent impact velocities with unclothed skin. A serious wound is arbitrarily defined as a laceration of the skin with missile penetration into the tissues to a depth of 1 cm (about 0.4 inch) or more. [ref. Table 12.47] X
- 5 In this connection, a casualty is defined as an individual so injured that he would probably be a burden on others. Some of the casualties would prove fatal, especially in the absence of medical care. [ref. § 12.49] X
- 6 The more general expression “ionizing radiations” is often employed instead of nuclear radiations, since this permits the inclusion of radiations of nonnuclear origin, e.g., X rays, having similar biological effects. [ref. § 12.91] X
- 7 Gamma rays from cobalt-60 have been commonly specified for this purpose. [ref. § 12.94] X
- 8 Beta particles from sources on or near the body can also cause skin lesions, called “beta burns” (§ 12.155 et seq.). [ref. § 12.97] X
- 9 The curves in Chapter VIII that show the neutron dose in rads at a particular location relative to a nuclear explosion calculated by considering the contributions of neutrons in various energy ranges at that location for typical weapons spectra. Multiplication of these doses by the appropriate RBE gives the corresponding biological dose in rems. [ref. § 12.97] X
- 10 The Atomic Bomb Casualty Commission (ABCC) of the U.S. National Academy of Sciences-Na tional Research Council was sponsored by the U.S. Atomic Energy Commission (now the Energy Research and Development Administration) and administered in cooperation with the Japanese National Institute of Health. One of its purposes was to study the long-term effects of human exposure to nuclear radiation. In 1975, the ABCC was superseded by the Radiation Effects Research Foundation which is supported equally by Japan and the United States. [ref. § 12.142] X
- 11 Even under these conditions, only about 10 percent of the strontium or barium is actually absorbed. [ref. § 12.169] X
- 12 Much valuable information on delayed fallout and related problems can be found in the published Hearings before the Special Committee on Radiation of the Joint Committee on Atomic Energy, Congress of the United States: “The Nature of Radioactive Fallout and its Effects on Man,” May 27 to June 7, 1957; “Fallout from Nuclear Weapons Tests,” May 5 to 8, 1959; and “Biological and Environmental Effects of Nuclear War,” June 22 to 26, 1959 (U.S. Government Printing Office). [ref. LONG-TERM HAZARD FROM DELAYED FALLOUT] X
- 13 Data from strontium-90 excretion by the Marshallese people and studies in a case of accidental inhalation indicate that for an acute intake the major portion of the absorbed strontium-90 is excreted with a biological half-life of 40 days during the first year. During the next 2 years, at least, a smaller fraction is excreted with a biological half-life of 500 days. The remaining portion (less than 10 percent) is tightly bound to bone and is excreted very slowly with a long biological half-life of about 50 years. In this latter case, the effective half-life (§ 12.170) would be about 18 years. The situation for a chronic intake e.g., from delayed fallout, although not the same, would be similar. [ref. § 12.188] X
- 14 The energy of the strontium-90 beta particles is 0.54 MeV. However, its daughter, yttrium-90, which has the short half-life of only 64 hours, emits 2.27-MeV beta particles (no gamma rays); the decay product is stable zirconium-90. Thus, both 0.54- and 2.27-MeV beta particles accompany the decay of strontium-90. (The energies quoted are maxima; the average energy is about one-third of the maximum.) [ref. § 12.189] X
- 15 It is to be expected that areas near the explosion will be more highly contaminated in strontium-90 than are more distant regions, to an extent dependent upon such factors as the height (or depth) of burst, the total and fission yields of the explosion, and the prevailing atmospheric conditions. Because of the phenomenon of fractionation, the proportion of strontium-90 in the local (early) fallout will generally be less than that in the worldwide (delayed) fallout. It is of interest to mention, too, that the strontium-90 in early fallout appears to be in a less soluble form, and hence probably less readily accessible to plants, than that present in the delayed fallout. [ref. § 12.195] X
- 16 In the experiments with gamma and X rays, measurements were made of exposures in roentgens per minute; hence. these units are used here. The dose rates in rads (or rems) per minute to the mouse gonads are probably essentially the same as the exposure rates. All conclusions concerning exposure-rate effects thus apply equally to dose-rate effects. [ref. § 12.210] X
- 17 Gamma rays accompanying the decay of cesium-137 (and of other species of moderately long half-life) deposited on the ground as delayed fallout, i.e.. as an external source, can make a significant contribution to the genetic dose. [ref. § 12.217] X
- 18 The more technical discussion in § 12.220 through § 12.239 may be omitted without loss of continuity. A general treatment of radiation effects on plants and farm animals is given in § 12.240 et seq. [ref. PATHOLOGY OF ACUTE RADIATION INJURY] X
- 19 In the tests, the gamma-ray exposures were measured in roentgens and so are expressed in this form in the table,The actual whole-body doses in rems would probably not be greatly different. [ref. § 12.249] X
- 20 The rumen is the first stomach (or pouch) of a ruminant and the abomasum is the fourth (or true) stomach. [ref. § 12.253] X